Loss of myeloid related protein-8/14 exacerbates cardiac allograft rejection
- PMID: 22144572
- PMCID: PMC3277828
- DOI: 10.1161/CIRCULATIONAHA.110.009910
Loss of myeloid related protein-8/14 exacerbates cardiac allograft rejection
Abstract
Background: The calcium-binding proteins myeloid-related protein (MRP)-8 (S100A8) and MRP-14 (S100A9) form MRP-8/14 heterodimers (S100A8/A9, calprotectin) that regulate myeloid cell function and inflammatory responses and serve as early serum markers for monitoring acute allograft rejection. Despite functioning as a proinflammatory mediator, the pathophysiological role of MRP-8/14 complexes in cardiovascular disease is incompletely defined. This study investigated the role of MRP-8/14 in cardiac allograft rejection using MRP-14(-/-) mice that lack MRP-8/14 complexes.
Methods and results: We examined parenchymal rejection after major histocompatibility complex class II allomismatched cardiac transplantation (bm12 donor heart and B6 recipients) in wild-type (WT) and MRP-14(-/-) recipients. Allograft survival averaged 5.9±2.9 weeks (n=10) in MRP-14(-/-) recipients compared with >12 weeks (n=15; P<0.0001) in WT recipients. Two weeks after transplantation, allografts in MRP-14(-/-) recipients had significantly higher parenchymal rejection scores (2.8±0.8; n=8) than did WT recipients (0.8±0.8; n=12; P<0.0001). Compared with WT recipients, allografts in MRP-14(-/-) recipients had significantly increased T-cell and macrophage infiltration and increased mRNA levels of interferon-γ and interferon-γ-associated chemokines (CXCL9, CXCL10, and CXCL11), interleukin-6, and interleukin-17 with significantly higher levels of Th17 cells. MRP-14(-/-) recipients also had significantly more lymphocytes in the adjacent para-aortic lymph nodes than did WT recipients (cells per lymph node: 23.7±0.7×10(5) for MRP-14(-/-) versus 6.0±0.2×10(5) for WT; P<0.0001). The dendritic cells (DCs) of the MRP-14(-/-) recipients of bm12 hearts expressed significantly higher levels of the costimulatory molecules CD80 and CD86 than did those of WT recipients 2 weeks after transplantation. Mixed leukocyte reactions with allo-endothelial cell-primed MRP-14(-/-) DCs resulted in significantly higher antigen-presenting function than reactions using WT DCs. Ovalbumin-primed MRP-14(-/-) DCs augmented proliferation of OT-II (ovalbumin-specific T cell receptor transgenic) CD4(+) T cells with increased interleukin-2 and interferon-γ production. Cardiac allografts of B6 major histocompatibility complex class II(-/-) hosts and of B6 WT hosts receiving MRP-14(-/-) DCs had significantly augmented inflammatory cell infiltration and accelerated allograft rejection compared with WT DCs from transferred recipient allografts. Bone marrow-derived MRP-14(-/-) DCs infected with MRP-8 and MRP-14 retroviral vectors showed significantly decreased CD80 and CD86 expression compared with controls, indicating that MRP-8/14 regulates B7-costimulatory molecule expression.
Conclusions: Our results indicate that MRP-14 regulates B7 molecule expression and reduces antigen presentation by DCs and subsequent T-cell priming. The absence of MRP-14 markedly increased T-cell activation and exacerbated allograft rejection, indicating a previously unrecognized role for MRP-14 in immune cell biology.
Conflict of interest statement
Figures
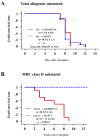
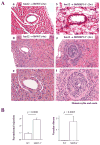
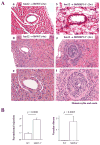
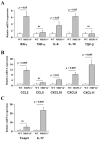
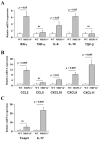
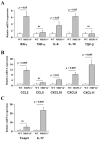
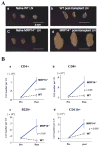
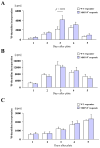
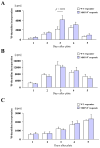
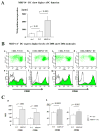
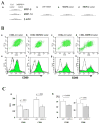
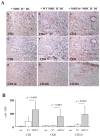
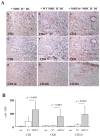
Similar articles
-
Leukocyte integrin Mac-1 promotes acute cardiac allograft rejection.Circulation. 2008 Apr 15;117(15):1997-2008. doi: 10.1161/CIRCULATIONAHA.107.724310. Epub 2008 Mar 31. Circulation. 2008. PMID: 18378617 Free PMC article.
-
Bone marrow-derived immature dendritic cells prime in vivo alloreactive T cells for interleukin-4-dependent rejection of major histocompatibility complex class II antigen-disparate cardiac allograft.Transplantation. 2003 Feb 15;75(3):407-13. doi: 10.1097/01.TP.0000044172.19087.22. Transplantation. 2003. PMID: 12589166
-
Monokine induced by interferon-gamma (MIG/CXCL9) is derived from both donor and recipient sources during rejection of class II major histocompatibility complex disparate skin allografts.Am J Pathol. 2009 Jun;174(6):2172-81. doi: 10.2353/ajpath.2009.080516. Epub 2009 Apr 23. Am J Pathol. 2009. PMID: 19389928 Free PMC article.
-
Dendritic Cell-Mediated Regulation of Liver Ischemia-Reperfusion Injury and Liver Transplant Rejection.Front Immunol. 2021 Jun 28;12:705465. doi: 10.3389/fimmu.2021.705465. eCollection 2021. Front Immunol. 2021. PMID: 34262574 Free PMC article. Review.
-
Endogenous memory T cells with donor-reactivity: early post-transplant mediators of acute graft injury in unsensitized recipients.Transpl Int. 2021 Aug;34(8):1360-1373. doi: 10.1111/tri.13900. Epub 2021 Jun 29. Transpl Int. 2021. PMID: 33963616 Free PMC article. Review.
Cited by
-
S100 proteins as diagnostic and prognostic markers in colorectal and hepatocellular carcinoma.Hepat Mon. 2012 Oct;12(10 HCC):e7240. doi: 10.5812/hepatmon.7240. Epub 2012 Oct 12. Hepat Mon. 2012. PMID: 23166536 Free PMC article.
-
Functional characterization of S100A8 and S100A9 in altering monolayer permeability of human umbilical endothelial cells.PLoS One. 2014 Mar 3;9(3):e90472. doi: 10.1371/journal.pone.0090472. eCollection 2014. PLoS One. 2014. PMID: 24595267 Free PMC article.
-
Myeloid-derived suppressor cells in the development of lung cancer.Cancer Immunol Res. 2014 Jan;2(1):50-8. doi: 10.1158/2326-6066.CIR-13-0129. Epub 2013 Oct 18. Cancer Immunol Res. 2014. PMID: 24778162 Free PMC article.
-
Serum inflammatory cytokines as disease biomarkers in the DE50-MD dog model of Duchenne muscular dystrophy.Dis Model Mech. 2022 Dec 1;15(12):dmm049394. doi: 10.1242/dmm.049394. Epub 2022 Dec 9. Dis Model Mech. 2022. PMID: 36444978 Free PMC article.
-
Resolution of Inflammation: What Controls Its Onset?Front Immunol. 2016 Apr 26;7:160. doi: 10.3389/fimmu.2016.00160. eCollection 2016. Front Immunol. 2016. PMID: 27199985 Free PMC article. Review.
References
-
- Edgeworth J, Gorman M, Bennett R, Freemont P, Hogg N. Identification of p8,14 as a highly abundant heterodimeric calcium binding protein complex of myeloid cells. J Biol Chem. 1991;266:7706–7713. - PubMed
-
- Hunter MJ, Chazin WJ. High level expression and dimer characterization of the S100 EF-hand proteins, migration inhibitory factor-related proteins 8 and 14. J Biol Chem. 1998;273:12427–12435. - PubMed
-
- Lagasse E, Weissman IL. Mouse MRP8 and MRP14, two intracellular calcium-binding proteins associated with the development of the myeloid lineage. Blood. 1992;79:1907–1915. - PubMed
-
- Odink K, Cerletti N, Bruggen J, Clerc RG, Tarcsay L, Zwadlo G, Gerhards G, Schlegel R, Sorg C. Two calcium-binding proteins in infiltrate macrophages of rheumatoid arthritis. Nature. 1987;330:80–82. - PubMed
-
- Nacken W, Sopalla C, Propper C, Sorg C, Kerkhoff C. Biochemical characterization of the murine S100A9 (MRP14) protein suggests that it is functionally equivalent to its human counterpart despite its low degree of sequence homology. Eur J Biochem. 2000;267:560–565. - PubMed
Publication types
MeSH terms
Substances
Grants and funding
- R37 HL057506/HL/NHLBI NIH HHS/United States
- R01 HL085816/HL/NHLBI NIH HHS/United States
- HL60942/HL/NHLBI NIH HHS/United States
- 1K08HL086672/HL/NHLBI NIH HHS/United States
- R01 HL034636-23/HL/NHLBI NIH HHS/United States
- HL-43364/HL/NHLBI NIH HHS/United States
- R01 HL085816-04/HL/NHLBI NIH HHS/United States
- HL57506/HL/NHLBI NIH HHS/United States
- K08 HL086672-05/HL/NHLBI NIH HHS/United States
- R01 HD043364/HD/NICHD NIH HHS/United States
- HL85816/HL/NHLBI NIH HHS/United States
- R01 HL034636/HL/NHLBI NIH HHS/United States
- K08 HL086672/HL/NHLBI NIH HHS/United States
- R01 HL043364-14/HL/NHLBI NIH HHS/United States
- R01 HL057506-10/HL/NHLBI NIH HHS/United States
- R37 HL034636/HL/NHLBI NIH HHS/United States
- R01 HL087282/HL/NHLBI NIH HHS/United States
- R01 HL057506/HL/NHLBI NIH HHS/United States
- R01 HL060942/HL/NHLBI NIH HHS/United States
- HL-34636/HL/NHLBI NIH HHS/United States
LinkOut - more resources
Full Text Sources
Medical
Molecular Biology Databases
Research Materials
Miscellaneous