Combined genetic inactivation of β2-Microglobulin and CD58 reveals frequent escape from immune recognition in diffuse large B cell lymphoma
- PMID: 22137796
- PMCID: PMC3660995
- DOI: 10.1016/j.ccr.2011.11.006
Combined genetic inactivation of β2-Microglobulin and CD58 reveals frequent escape from immune recognition in diffuse large B cell lymphoma
Abstract
We report that diffuse large B cell lymphoma (DLBCL) commonly fails to express cell-surface molecules necessary for the recognition of tumor cells by immune-effector cells. In 29% of cases, mutations and deletions inactivate the β2-Microglobulin gene, thus preventing the cell-surface expression of the HLA class-I (HLA-I) complex that is necessary for recognition by CD8(+) cytotoxic T cells. In 21% of cases, analogous lesions involve the CD58 gene, which encodes a molecule involved in T and natural killer cell-mediated responses. In addition to gene inactivation, alternative mechanisms lead to aberrant expression of HLA-I and CD58 in >60% of DLBCL. These two events are significantly associated in this disease, suggesting that they are coselected during lymphomagenesis for their combined role in escape from immune-surveillance.
Copyright © 2011 Elsevier Inc. All rights reserved.
Figures
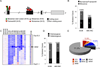
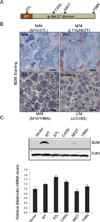
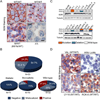
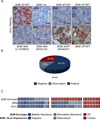
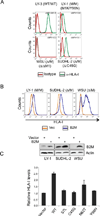
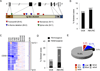
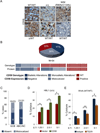
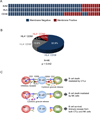
Similar articles
-
Genetic mechanisms of HLA-I loss and immune escape in diffuse large B cell lymphoma.Proc Natl Acad Sci U S A. 2021 Jun 1;118(22):e2104504118. doi: 10.1073/pnas.2104504118. Proc Natl Acad Sci U S A. 2021. PMID: 34050029 Free PMC article.
-
CD58 Alterations Govern Antitumor Immune Responses by Inducing PDL1 and IDO in Diffuse Large B-Cell Lymphoma.Cancer Res. 2024 Jul 2;84(13):2123-2140. doi: 10.1158/0008-5472.CAN-23-2874. Cancer Res. 2024. PMID: 38635903
-
Beta2-microglobulin aberrations in diffuse large B-cell lymphoma of the testis and the central nervous system.Int J Cancer. 2003 Jan 20;103(3):393-8. doi: 10.1002/ijc.10824. Int J Cancer. 2003. PMID: 12471623
-
Beta2-microglobulin gene mutations in human melanoma cells: molecular characterization and implications for immune surveillance.Melanoma Res. 1997 Aug;7 Suppl 2:S67-74. Melanoma Res. 1997. PMID: 9578419 Review.
-
Mutational landscape of immune surveillance genes in diffuse large B-cell lymphoma.Expert Rev Hematol. 2020 Jun;13(6):655-668. doi: 10.1080/17474086.2020.1755958. Epub 2020 Apr 27. Expert Rev Hematol. 2020. PMID: 32293210 Review.
Cited by
-
[The progress of diagnostic and prognostic molecular markers of lymphoma].Zhonghua Xue Ye Xue Za Zhi. 2015 Apr;36(4):354-7. doi: 10.3760/cma.j.issn.0253-2727.2015.04.022. Zhonghua Xue Ye Xue Za Zhi. 2015. PMID: 25916304 Free PMC article. Chinese. No abstract available.
-
Integrative analysis reveals an outcome-associated and targetable pattern of p53 and cell cycle deregulation in diffuse large B cell lymphoma.Cancer Cell. 2012 Sep 11;22(3):359-72. doi: 10.1016/j.ccr.2012.07.014. Cancer Cell. 2012. PMID: 22975378 Free PMC article.
-
Harnessing natural killer cells for refractory/relapsed non-Hodgkin lymphoma: biological roles, clinical trials, and future prospective.Biomark Res. 2024 Jul 17;12(1):66. doi: 10.1186/s40364-024-00610-z. Biomark Res. 2024. PMID: 39020411 Free PMC article. Review.
-
Treatment resistance in diffuse large B-cell lymphoma.Leukemia. 2021 Aug;35(8):2151-2165. doi: 10.1038/s41375-021-01285-3. Epub 2021 May 20. Leukemia. 2021. PMID: 34017074 Review.
-
Oncogenic Rag GTPase signaling enhances B cell activation and drives follicular lymphoma sensitive to pharmacological inhibition of mTOR.Nat Metab. 2019 Aug;1(8):775-789. doi: 10.1038/s42255-019-0098-8. Epub 2019 Aug 19. Nat Metab. 2019. PMID: 31579886 Free PMC article.
References
-
- Abramson JS, Shipp MA. Advances in the biology and therapy of diffuse large B-cell lymphoma: moving toward a molecularly targeted approach. Blood. 2005;106:1164–1174. - PubMed
-
- Altomonte M, Gloghini A, Bertola G, Gasparollo A, Carbone A, Ferrone S, Maio M. Differential expression of cell adhesion molecules CD54/CD11a and CD58/CD2 by human melanoma cells and functional role in their interaction with cytotoxic cells. Cancer Res. 1993;53:3343–3348. - PubMed
-
- Billaud M, Rousset F, Calender A, Cordier M, Aubry JP, Laisse V, Lenoir GM. Low expression of lymphocyte function-associated antigen (LFA)-1 and LFA-3 adhesion molecules is a common trait in Burkitt's lymphoma associated with and not associated with Epstein-Barr virus. Blood. 1990;75:1827–1833. - PubMed
-
- Bjorkman PJ, Saper MA, Samraoui B, Bennett WS, Strominger JL, Wiley DC. Structure of the human class I histocompatibility antigen, HLA-A2. Nature. 1987;329:506–512. - PubMed
-
- Bolhuis RL, Roozemond RC, van de Griend RJ. Induction and blocking of cytolysis in CD2+, CD3− NK and CD2+, CD3+ cytotoxic T lymphocytes via CD2 50 KD sheep erythrocyte receptor. J Immunol. 1986;136:3939–3944. - PubMed
Publication types
MeSH terms
Substances
Associated data
- Actions
Grants and funding
LinkOut - more resources
Full Text Sources
Other Literature Sources
Molecular Biology Databases
Research Materials