Heat shock factor binding in Alu repeats expands its involvement in stress through an antisense mechanism
- PMID: 22112862
- PMCID: PMC3334603
- DOI: 10.1186/gb-2011-12-11-r117
Heat shock factor binding in Alu repeats expands its involvement in stress through an antisense mechanism
Abstract
Background: Alu RNAs are present at elevated levels in stress conditions and, consequently, Alu repeats are increasingly being associated with the physiological stress response. Alu repeats are known to harbor transcription factor binding sites that modulate RNA pol II transcription and Alu RNAs act as transcriptional co-repressors through pol II binding in the promoter regions of heat shock responsive genes. An observation of a putative heat shock factor (HSF) binding site in Alu led us to explore whether, through HSF binding, these elements could further contribute to the heat shock response repertoire.
Results: Alu density was significantly enriched in transcripts that are down-regulated following heat shock recovery in HeLa cells. ChIP analysis confirmed HSF binding to a consensus motif exhibiting positional conservation across various Alu subfamilies, and reporter constructs demonstrated a sequence-specific two-fold induction of these sites in response to heat shock. These motifs were over-represented in the genic regions of down-regulated transcripts in antisense oriented Alus. Affymetrix Exon arrays detected antisense signals in a significant fraction of the down-regulated transcripts, 50% of which harbored HSF sites within 5 kb. siRNA knockdown of the selected antisense transcripts led to the over-expression, following heat shock, of their corresponding down-regulated transcripts. The antisense transcripts were significantly enriched in processes related to RNA pol III transcription and the TFIIIC complex.
Conclusions: We demonstrate a non-random presence of Alu repeats harboring HSF sites in heat shock responsive transcripts. This presence underlies an antisense-mediated mechanism that represents a novel component of Alu and HSF involvement in the heat shock response.
Figures
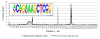
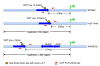
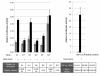
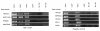
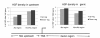
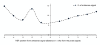
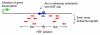
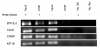
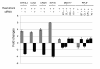
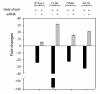
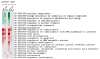
Similar articles
-
Combined sense-antisense Alu elements activate the EGFP reporter gene when stable transfection.Mol Genet Genomics. 2017 Aug;292(4):833-846. doi: 10.1007/s00438-017-1312-6. Epub 2017 Mar 29. Mol Genet Genomics. 2017. PMID: 28357596
-
Human Alu RNA is a modular transacting repressor of mRNA transcription during heat shock.Mol Cell. 2008 Feb 29;29(4):499-509. doi: 10.1016/j.molcel.2007.12.013. Mol Cell. 2008. PMID: 18313387
-
Evolution and distribution of RNA polymerase II regulatory sites from RNA polymerase III dependant mobile Alu elements.BMC Evol Biol. 2004 Oct 4;4:37. doi: 10.1186/1471-2148-4-37. BMC Evol Biol. 2004. PMID: 15461819 Free PMC article.
-
From 'JUNK' to just unexplored noncoding knowledge: the case of transcribed Alus.Brief Funct Genomics. 2011 Sep;10(5):294-311. doi: 10.1093/bfgp/elr029. Brief Funct Genomics. 2011. PMID: 21987713 Review.
-
ALUternative Regulation for Gene Expression.Trends Cell Biol. 2017 Jul;27(7):480-490. doi: 10.1016/j.tcb.2017.01.002. Epub 2017 Feb 10. Trends Cell Biol. 2017. PMID: 28209295 Review.
Cited by
-
Impact of heat shock transcription factor 1 on global gene expression profiles in cells which induce either cytoprotective or pro-apoptotic response following hyperthermia.BMC Genomics. 2013 Jul 8;14:456. doi: 10.1186/1471-2164-14-456. BMC Genomics. 2013. PMID: 23834426 Free PMC article.
-
Not All Stressors Are Equal: Mechanism of Stressors on RPE Cell Degeneration.Front Cell Dev Biol. 2020 Nov 19;8:591067. doi: 10.3389/fcell.2020.591067. eCollection 2020. Front Cell Dev Biol. 2020. PMID: 33330470 Free PMC article. Review.
-
HSF1-Activated Non-Coding Stress Response: Satellite lncRNAs and Beyond, an Emerging Story with a Complex Scenario.Genes (Basel). 2022 Mar 27;13(4):597. doi: 10.3390/genes13040597. Genes (Basel). 2022. PMID: 35456403 Free PMC article. Review.
-
Transcriptome-wide expansion of non-coding regulatory switches: evidence from co-occurrence of Alu exonization, antisense and editing.Nucleic Acids Res. 2013 Feb 1;41(4):2121-37. doi: 10.1093/nar/gks1457. Epub 2013 Jan 8. Nucleic Acids Res. 2013. PMID: 23303787 Free PMC article.
-
The CLN3 gene and protein: What we know.Mol Genet Genomic Med. 2019 Dec;7(12):e859. doi: 10.1002/mgg3.859. Epub 2019 Sep 30. Mol Genet Genomic Med. 2019. PMID: 31568712 Free PMC article. Review.
References
-
- Cotnoir-White D, Laperriere D, Mader S. Evolution of the repertoire of nuclear receptor binding sites in genomes. Mol Cell Endocrinol. 2011;334:76–82. - PubMed
Publication types
MeSH terms
Substances
Associated data
- Actions
- Actions
LinkOut - more resources
Full Text Sources
Molecular Biology Databases