A microfabricated platform to measure and manipulate the mechanics of engineered cardiac microtissues
- PMID: 22092279
- PMCID: PMC3338105
- DOI: 10.1089/ten.tea.2011.0341
A microfabricated platform to measure and manipulate the mechanics of engineered cardiac microtissues
Abstract
Engineered myocardial tissues can be used to elucidate fundamental features of myocardial biology, develop organotypic in vitro model systems, and as engineered tissue constructs for replacing damaged heart tissue in vivo. However, a key limitation is an inability to test the wide range of parameters (cell source, mechanical, soluble and electrical stimuli) that might impact the engineered tissue in a high-throughput manner and in an environment that mimics native heart tissue. Here we used microelectromechanical systems technology to generate arrays of cardiac microtissues (CMTs) embedded within three-dimensional micropatterned matrices. Microcantilevers simultaneously constrain CMT contraction and report forces generated by the CMTs in real time. We demonstrate the ability to routinely produce ~200 CMTs per million cardiac cells (<1 neonatal rat heart) whose spontaneous contraction frequency, duration, and forces can be tracked. Independently varying the mechanical stiffness of the cantilevers and collagen matrix revealed that both the dynamic force of cardiac contraction as well as the basal static tension within the CMT increased with boundary or matrix rigidity. Cell alignment is, however, reduced within a stiff collagen matrix; therefore, despite producing higher force, CMTs constructed from higher density collagen have a lower cross-sectional stress than those constructed from lower density collagen. We also study the effect of electrical stimulation on cell alignment and force generation within CMTs and we show that the combination of electrical stimulation and auxotonic load strongly improves both the structure and the function of the CMTs. Finally, we demonstrate the suitability of our technique for high-throughput monitoring of drug-induced changes in spontaneous frequency or contractility in CMTs as well as high-speed imaging of calcium dynamics using fluorescent dyes. Together, these results highlight the potential for this approach to quantitatively demonstrate the impact of physical parameters on the maturation, structure, and function of cardiac tissue and open the possibility to use high-throughput, low volume screening for studies on engineered myocardium.
Figures
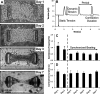
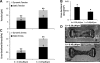
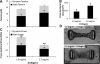
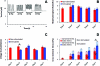
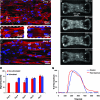
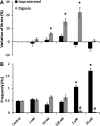
Similar articles
-
Microfabrication of a platform to measure and manipulate the mechanics of engineered microtissues.Methods Cell Biol. 2014;121:191-211. doi: 10.1016/B978-0-12-800281-0.00013-0. Methods Cell Biol. 2014. PMID: 24560511
-
Microfabricated tissue gauges to measure and manipulate forces from 3D microtissues.Proc Natl Acad Sci U S A. 2009 Jun 23;106(25):10097-102. doi: 10.1073/pnas.0900174106. Epub 2009 Jun 16. Proc Natl Acad Sci U S A. 2009. PMID: 19541627 Free PMC article.
-
Long-term contractile activity and thyroid hormone supplementation produce engineered rat myocardium with adult-like structure and function.Acta Biomater. 2018 Sep 15;78:98-110. doi: 10.1016/j.actbio.2018.08.003. Epub 2018 Aug 4. Acta Biomater. 2018. PMID: 30086384 Free PMC article.
-
Three-dimensional scaffold-free microtissues engineered for cardiac repair.J Mater Chem B. 2020 Sep 14;8(34):7571-7590. doi: 10.1039/d0tb01528h. Epub 2020 Jul 29. J Mater Chem B. 2020. PMID: 32724973 Free PMC article. Review.
-
Electrical and mechanical stimulation of cardiac cells and tissue constructs.Adv Drug Deliv Rev. 2016 Jan 15;96:135-55. doi: 10.1016/j.addr.2015.07.009. Epub 2015 Jul 30. Adv Drug Deliv Rev. 2016. PMID: 26232525 Free PMC article. Review.
Cited by
-
Aligned human cardiac syncytium for in vitro analysis of electrical, structural, and mechanical readouts.Biotechnol Bioeng. 2021 Jan;118(1):442-452. doi: 10.1002/bit.27582. Epub 2020 Oct 13. Biotechnol Bioeng. 2021. PMID: 32990953 Free PMC article.
-
Induced pluripotent stem cell-derived vascular smooth muscle cells.Vasc Biol. 2019 Dec 12;2(1):R1-R15. doi: 10.1530/VB-19-0028. eCollection 2020. Vasc Biol. 2019. PMID: 32923972 Free PMC article. Review.
-
A microfabricated magnetic actuation device for mechanical conditioning of arrays of 3D microtissues.Lab Chip. 2015 Jun 7;15(11):2496-503. doi: 10.1039/c4lc01395f. Epub 2015 May 11. Lab Chip. 2015. PMID: 25959132 Free PMC article.
-
Genetic and Tissue Engineering Approaches to Modeling the Mechanics of Human Heart Failure for Drug Discovery.Front Cardiovasc Med. 2018 Sep 19;5:120. doi: 10.3389/fcvm.2018.00120. eCollection 2018. Front Cardiovasc Med. 2018. PMID: 30283789 Free PMC article. Review.
-
Advancing functional engineered cardiac tissues toward a preclinical model of human myocardium.FASEB J. 2014 Feb;28(2):644-54. doi: 10.1096/fj.13-228007. Epub 2013 Oct 30. FASEB J. 2014. PMID: 24174427 Free PMC article.
References
-
- Mathers C.D. Bernard C. Iburg K.M. Inoue M. Ma Fat D. Shibuya K. Stein C. Tomijima N. Xu H. Global Burden of Disease: Data Sources, Methods and Results. World Health Organization; Geneva, Switzerland: 2008.
-
- Eschenhagen T. Zimmermann W.H. Engineering myocardial tissue. Circ Res. 2005;97:1220. - PubMed
-
- Carrier R.L. Papadaki M. Rupnick M. Schoen F.J. Bursac N. Langer R. Freed L.E. Vunjak-Novakovic G. Cardiac tissue engineering: cell seeding, cultivation parameters, and tissue construct characterization. Biotechnol Bioeng. 1999;64:580. - PubMed
-
- van Luyn M.J.A. Tio R.A. Gallego y van Seijen X.J. Plantinga J.A. de Leij L.F.M.H. DeJongste M.J.L. van Wachem P.B. Cardiac tissue engineering: characteristics of in unison contracting two- and three-dimensional neonatal rat ventricle cell (co)-cultures. Biomaterials. 2002;23:4793. - PubMed
-
- Hansen A. Eder A. Bonstrup M. Flato M. Mewe M. Schaaf S. Aksehirlioglu B. Schworer A. Uebeler J. Eschenhagen T. Development of a drug screening platform based on engineered heart. Tissue Circ Res. 2010;107:35. - PubMed
Publication types
MeSH terms
Grants and funding
LinkOut - more resources
Full Text Sources
Other Literature Sources