Th17 lymphocytes traffic to the central nervous system independently of α4 integrin expression during EAE
- PMID: 22025301
- PMCID: PMC3256959
- DOI: 10.1084/jem.20110434
Th17 lymphocytes traffic to the central nervous system independently of α4 integrin expression during EAE
Abstract
The integrin α4β1 (VLA-4) is used by encephalitogenic T cells to enter the central nervous system (CNS). However, both Th1 and Th17 cells are capable of inducing experimental autoimmune encephalomyelitis (EAE), and the molecular cues mediating the infiltration of Th1 versus Th17 cells into the CNS have not yet been defined. We investigated how blocking of α4 integrins affected trafficking of Th1 and Th17 cells into the CNS during EAE. Although antibody-mediated inhibition of α4 integrins prevented EAE when MOG(35-55)-specific Th1 cells were adoptively transferred, Th17 cells entered the brain, but not the spinal cord parenchyma, irrespective of α4 blockade. Accordingly, T cell-conditional α4-deficient mice were not resistant to actively induced EAE but showed an ataxic syndrome with predominantly supraspinal infiltrates of IL-23R(+)CCR6(+)CD4(+) T cells. The entry of α4-deficient Th17 cells into the CNS was abolished by blockade of LFA-1 (αLβ2 integrin). Thus, Th1 cells preferentially infiltrate the spinal cord via an α4 integrin-mediated mechanism, whereas the entry of Th17 cells into the brain parenchyma occurs in the absence of α4 integrins but is dependent on the expression of αLβ2. These observations have implications for the understanding of lesion localization, immunosurveillance, and drug design in multiple sclerosis.
Figures
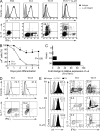
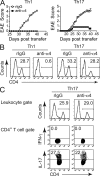
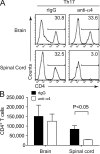
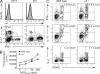
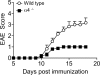
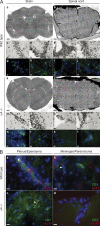
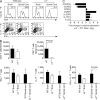
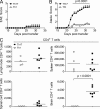
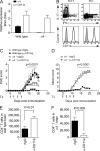
Similar articles
-
LFA-1 Controls Th1 and Th17 Motility Behavior in the Inflamed Central Nervous System.Front Immunol. 2019 Oct 18;10:2436. doi: 10.3389/fimmu.2019.02436. eCollection 2019. Front Immunol. 2019. PMID: 31681316 Free PMC article.
-
Alpha4 beta7 integrin controls Th17 cell trafficking in the spinal cord leptomeninges during experimental autoimmune encephalomyelitis.Front Immunol. 2023 Apr 18;14:1071553. doi: 10.3389/fimmu.2023.1071553. eCollection 2023. Front Immunol. 2023. PMID: 37143680 Free PMC article.
-
α4-integrin deficiency in B cells does not affect disease in a T-cell-mediated EAE disease model.Neurol Neuroimmunol Neuroinflamm. 2019 Apr 16;6(4):e563. doi: 10.1212/NXI.0000000000000563. eCollection 2019 Jul. Neurol Neuroimmunol Neuroinflamm. 2019. PMID: 31086806 Free PMC article.
-
Using EAE to better understand principles of immune function and autoimmune pathology.J Autoimmun. 2013 Sep;45:31-9. doi: 10.1016/j.jaut.2013.06.008. Epub 2013 Jul 9. J Autoimmun. 2013. PMID: 23849779 Free PMC article. Review.
-
Neuroinflammation at single cell level: What is new?J Leukoc Biol. 2020 Oct;108(4):1129-1137. doi: 10.1002/JLB.3MR0620-035R. Epub 2020 Aug 10. J Leukoc Biol. 2020. PMID: 32779279 Review.
Cited by
-
Asthma and posttraumatic stress disorder (PTSD): Emerging links, potential models and mechanisms.Brain Behav Immun. 2021 Oct;97:275-285. doi: 10.1016/j.bbi.2021.06.001. Epub 2021 Jun 6. Brain Behav Immun. 2021. PMID: 34107349 Free PMC article. Review.
-
T Cells as an Emerging Target for Chronic Pain Therapy.Front Mol Neurosci. 2019 Sep 11;12:216. doi: 10.3389/fnmol.2019.00216. eCollection 2019. Front Mol Neurosci. 2019. PMID: 31572125 Free PMC article.
-
IFN-γ is a therapeutic target in paraneoplastic cerebellar degeneration.JCI Insight. 2019 Apr 4;4(7):e127001. doi: 10.1172/jci.insight.127001. eCollection 2019 Apr 4. JCI Insight. 2019. PMID: 30944244 Free PMC article.
-
Intercellular adhesion molecule 1 mediates migration of Th1 and Th17 cells across human retinal vascular endothelium.Invest Ophthalmol Vis Sci. 2013 Oct 23;54(10):6917-25. doi: 10.1167/iovs.13-12058. Invest Ophthalmol Vis Sci. 2013. PMID: 24022011 Free PMC article.
-
Developmental endothelial locus-1 is a homeostatic factor in the central nervous system limiting neuroinflammation and demyelination.Mol Psychiatry. 2015 Jul;20(7):880-888. doi: 10.1038/mp.2014.146. Epub 2014 Nov 11. Mol Psychiatry. 2015. PMID: 25385367 Free PMC article.
References
-
- Barnett M., Prineas J., Buckland M., Parratt J., Pollard J. 2011. Massive astrocyte destruction in neuromyelitis optica despite natalizumab therapy. Mult. Scler. - PubMed
-
- Bartholomäus I., Kawakami N., Odoardi F., Schläger C., Miljkovic D., Ellwart J.W., Klinkert W.E., Flügel-Koch C., Issekutz T.B., Wekerle H., Flügel A. 2009. Effector T cell interactions with meningeal vascular structures in nascent autoimmune CNS lesions. Nature. 462:94–98 10.1038/nature08478 - DOI - PubMed
Publication types
MeSH terms
Substances
LinkOut - more resources
Full Text Sources
Other Literature Sources
Molecular Biology Databases
Research Materials