The same periplasmic ExbD residues mediate in vivo interactions between ExbD homodimers and ExbD-TonB heterodimers
- PMID: 21984795
- PMCID: PMC3232860
- DOI: 10.1128/JB.06190-11
The same periplasmic ExbD residues mediate in vivo interactions between ExbD homodimers and ExbD-TonB heterodimers
Abstract
The TonB system couples cytoplasmic membrane proton motive force to TonB-gated outer membrane transporters for active transport of nutrients into the periplasm. In Escherichia coli, cytoplasmic membrane proteins ExbB and ExbD promote conformational changes in TonB, which transmits this energy to the transporters. The only known energy-dependent interaction occurs between the periplasmic domains of TonB and ExbD. This study identified sites of in vivo homodimeric interactions within ExbD periplasmic domain residues 92 to 121. ExbD was active as a homodimer (ExbD(2)) but not through all Cys substitution sites, suggesting the existence of conformationally dynamic regions in the ExbD periplasmic domain. A subset of homodimeric interactions could not be modeled on the nuclear magnetic resonance (NMR) structure without significant distortion. Most importantly, the majority of ExbD Cys substitutions that mediated homodimer formation also mediated ExbD-TonB heterodimer formation with TonB A150C. Consistent with the implied competition, ExbD homodimer formation increased in the absence of TonB. Although ExbD D25 was not required for their formation, ExbD dimers interacted in vivo with ExbB. ExbD-TonB interactions required ExbD transmembrane domain residue D25. These results suggested a model where ExbD(2) assembled with ExbB undergoes a transmembrane domain-dependent transition and exchanges partners in localized homodimeric interfaces to form an ExbD(2)-TonB heterotrimer. The findings here were also consistent with our previous hypothesis that ExbD guides the conformation of the TonB periplasmic domain, which itself is conformationally dynamic.
Figures
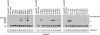
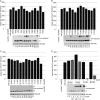
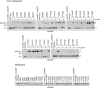
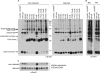
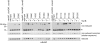
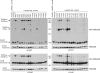
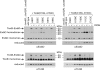
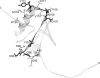
Similar articles
-
Identification of functionally important TonB-ExbD periplasmic domain interactions in vivo.J Bacteriol. 2012 Jun;194(12):3078-87. doi: 10.1128/JB.00018-12. Epub 2012 Apr 6. J Bacteriol. 2012. PMID: 22493017 Free PMC article.
-
The ExbD periplasmic domain contains distinct functional regions for two stages in TonB energization.J Bacteriol. 2012 Jun;194(12):3069-77. doi: 10.1128/JB.00015-12. Epub 2012 Apr 6. J Bacteriol. 2012. PMID: 22493019 Free PMC article.
-
Cytoplasmic membrane protonmotive force energizes periplasmic interactions between ExbD and TonB.Mol Microbiol. 2009 Aug;73(3):466-81. doi: 10.1111/j.1365-2958.2009.06785.x. Epub 2009 Jul 16. Mol Microbiol. 2009. PMID: 19627500 Free PMC article.
-
Energization of Outer Membrane Transport by the ExbB ExbD Molecular Motor.J Bacteriol. 2023 Jun 27;205(6):e0003523. doi: 10.1128/jb.00035-23. Epub 2023 May 23. J Bacteriol. 2023. PMID: 37219427 Free PMC article. Review.
-
Analysis of the Escherichia coli Tol-Pal and TonB systems by periplasmic production of Tol, TonB, colicin, or phage capsid soluble domains.Biochimie. 2002 May-Jun;84(5-6):413-21. doi: 10.1016/s0300-9084(02)01423-2. Biochimie. 2002. PMID: 12423784 Review.
Cited by
-
A new class of biological ion-driven rotary molecular motors with 5:2 symmetry.Front Microbiol. 2022 Aug 5;13:948383. doi: 10.3389/fmicb.2022.948383. eCollection 2022. Front Microbiol. 2022. PMID: 35992645 Free PMC article. Review.
-
Structure and Stoichiometry of the Ton Molecular Motor.Int J Mol Sci. 2020 Jan 7;21(2):375. doi: 10.3390/ijms21020375. Int J Mol Sci. 2020. PMID: 31936081 Free PMC article. Review.
-
Identification of functionally important TonB-ExbD periplasmic domain interactions in vivo.J Bacteriol. 2012 Jun;194(12):3078-87. doi: 10.1128/JB.00018-12. Epub 2012 Apr 6. J Bacteriol. 2012. PMID: 22493017 Free PMC article.
-
Cryo-EM structure of the bacterial Ton motor subcomplex ExbB-ExbD provides information on structure and stoichiometry.Commun Biol. 2019 Oct 4;2:358. doi: 10.1038/s42003-019-0604-2. eCollection 2019. Commun Biol. 2019. PMID: 31602407 Free PMC article.
-
Membrane Protein Complex ExbB4-ExbD1-TonB1 from Escherichia coli Demonstrates Conformational Plasticity.J Bacteriol. 2015 Jun;197(11):1873-85. doi: 10.1128/JB.00069-15. Epub 2015 Mar 23. J Bacteriol. 2015. PMID: 25802296 Free PMC article.
References
-
- Braun V., Herrmann C. 1993. Evolutionary relationship of uptake systems for biopolymers in Escherichia coli: cross-complementation between the TonB-ExbB-ExbD and the TolA-TolQ-TolR proteins. Mol. Microbiol. 8:261–268 - PubMed
Publication types
MeSH terms
Substances
Grants and funding
LinkOut - more resources
Full Text Sources
Molecular Biology Databases