Grafted human-induced pluripotent stem-cell-derived neurospheres promote motor functional recovery after spinal cord injury in mice
- PMID: 21949375
- PMCID: PMC3189018
- DOI: 10.1073/pnas.1108077108
Grafted human-induced pluripotent stem-cell-derived neurospheres promote motor functional recovery after spinal cord injury in mice
Abstract
Once their safety is confirmed, human-induced pluripotent stem cells (hiPSCs), which do not entail ethical concerns, may become a preferred cell source for regenerative medicine. Here, we investigated the therapeutic potential of transplanting hiPSC-derived neurospheres (hiPSC-NSs) into nonobese diabetic (NOD)-severe combined immunodeficient (SCID) mice to treat spinal cord injury (SCI). For this, we used a hiPSC clone (201B7), established by transducing four reprogramming factors (Oct3/4, Sox2, Klf4, and c-Myc) into adult human fibroblasts. Grafted hiPSC-NSs survived, migrated, and differentiated into the three major neural lineages (neurons, astrocytes, and oligodendrocytes) within the injured spinal cord. They showed both cell-autonomous and noncell-autonomous (trophic) effects, including synapse formation between hiPSC-NS-derived neurons and host mouse neurons, expression of neurotrophic factors, angiogenesis, axonal regrowth, and increased amounts of myelin in the injured area. These positive effects resulted in significantly better functional recovery compared with vehicle-treated control animals, and the recovery persisted through the end of the observation period, 112 d post-SCI. No tumor formation was observed in the hiPSC-NS-grafted mice. These findings suggest that hiPSCs give rise to neural stem/progenitor cells that support improved function post-SCI and are a promising cell source for its treatment.
Conflict of interest statement
The authors declare no conflict of interest.
Figures
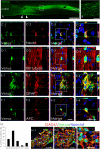
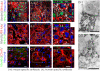
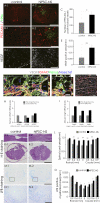
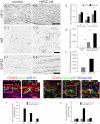
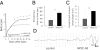
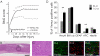
Similar articles
-
Pre-evaluated safe human iPSC-derived neural stem cells promote functional recovery after spinal cord injury in common marmoset without tumorigenicity.PLoS One. 2012;7(12):e52787. doi: 10.1371/journal.pone.0052787. Epub 2012 Dec 27. PLoS One. 2012. PMID: 23300777 Free PMC article.
-
[Regeneration of the central nervous system using iPS cell-technologies].Rinsho Shinkeigaku. 2009 Nov;49(11):825-6. doi: 10.5692/clinicalneurol.49.825. Rinsho Shinkeigaku. 2009. PMID: 20030221 Review. Japanese.
-
Therapeutic potential of appropriately evaluated safe-induced pluripotent stem cells for spinal cord injury.Proc Natl Acad Sci U S A. 2010 Jul 13;107(28):12704-9. doi: 10.1073/pnas.0910106107. Epub 2010 Jul 6. Proc Natl Acad Sci U S A. 2010. PMID: 20615974 Free PMC article.
-
Therapeutic potential of induced neural stem cells for spinal cord injury.J Biol Chem. 2014 Nov 21;289(47):32512-25. doi: 10.1074/jbc.M114.588871. Epub 2014 Oct 6. J Biol Chem. 2014. PMID: 25294882 Free PMC article.
-
[Therapeutic potential of induced pluripotent stem cells for spinal cord injury].Brain Nerve. 2012 Jan;64(1):17-27. Brain Nerve. 2012. PMID: 22223498 Review. Japanese.
Cited by
-
Human embryonic stem cell-derived oligodendrocyte progenitors aid in functional recovery of sensory pathways following contusive spinal cord injury.PLoS One. 2012;7(10):e47645. doi: 10.1371/journal.pone.0047645. Epub 2012 Oct 16. PLoS One. 2012. PMID: 23091637 Free PMC article.
-
Hydrogels and Cell Based Therapies in Spinal Cord Injury Regeneration.Stem Cells Int. 2015;2015:948040. doi: 10.1155/2015/948040. Epub 2015 Jun 1. Stem Cells Int. 2015. PMID: 26124844 Free PMC article. Review.
-
Translation of Human-Induced Pluripotent Stem Cells: From Clinical Trial in a Dish to Precision Medicine.J Am Coll Cardiol. 2016 May 10;67(18):2161-2176. doi: 10.1016/j.jacc.2016.01.083. J Am Coll Cardiol. 2016. PMID: 27151349 Free PMC article. Review.
-
Cell therapy for neurological disorders.Nat Med. 2024 Oct;30(10):2756-2770. doi: 10.1038/s41591-024-03281-3. Epub 2024 Oct 15. Nat Med. 2024. PMID: 39407034 Review.
-
Neuronal Replacement in Stem Cell Therapy for Stroke: Filling the Gap.Front Cell Dev Biol. 2021 Apr 6;9:662636. doi: 10.3389/fcell.2021.662636. eCollection 2021. Front Cell Dev Biol. 2021. PMID: 33889578 Free PMC article. Review.
References
-
- Björklund A, Lindvall O. Cell replacement therapies for central nervous system disorders. Nat Neurosci. 2000;3:537–544. - PubMed
-
- Gage FH. Mammalian neural stem cells. Science. 2000;287:1433–1438. - PubMed
-
- Lindvall O, Kokaia Z. Stem cells for the treatment of neurological disorders. Nature. 2006;441:1094–1096. - PubMed
Publication types
MeSH terms
LinkOut - more resources
Full Text Sources
Other Literature Sources
Medical
Molecular Biology Databases
Miscellaneous