Gene-specific transcription activation via long-range allosteric shape-shifting
- PMID: 21916844
- PMCID: PMC7385929
- DOI: 10.1042/BJ20110972
Gene-specific transcription activation via long-range allosteric shape-shifting
Abstract
How is specificity transmitted over long distances at the molecular level? REs (regulatory elements) are often far from transcription start sites. In the present review we discuss possible mechanisms to explain how information from specific REs is conveyed to the basal transcription machinery through TFs (transcription factors) and the Mediator complex. We hypothesize that this occurs through allosteric pathways: binding of a TF to a RE results in changes in the AD (activation domain) of the TF, which binds to Mediator and alters the distribution of the Mediator conformations, thereby affecting transcription initiation/activation. We argue that Mediator is formed by highly disordered proteins with large densely packed interfaces that make efficient long-range signal propagation possible. We suggest two possible general mechanisms for Mediator action: one in which Mediator influences PIC (pre-initiation complex) assembly and transcription initiation, and another in which Mediator exerts its effect on the already assembled but stalled transcription complex. We summarize (i) relevant information from the literature about Mediator composition, organization and structure; (ii) Mediator interaction partners and their effect on Mediator conformation, function and correlation to the RNA Pol II (polymerase II) CTD (C-terminal domain) phosphorylation; and (iii) propose that different allosteric signal propagation pathways in Mediator relate to PIC assembly and polymerase activation of the stalled transcription complex. The emerging picture provides for the first time a mechanistic view of allosteric signalling from the RE sequence to transcription activation, and an insight into how gene specificity and signal transmission can take place in transcription initiation.
Figures
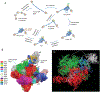
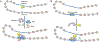
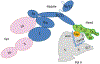
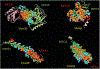
Similar articles
-
The Mediator complex and transcription regulation.Crit Rev Biochem Mol Biol. 2013 Nov-Dec;48(6):575-608. doi: 10.3109/10409238.2013.840259. Epub 2013 Oct 3. Crit Rev Biochem Mol Biol. 2013. PMID: 24088064 Free PMC article. Review.
-
Mediator, TATA-binding protein, and RNA polymerase II contribute to low histone occupancy at active gene promoters in yeast.J Biol Chem. 2014 May 23;289(21):14981-95. doi: 10.1074/jbc.M113.529354. Epub 2014 Apr 11. J Biol Chem. 2014. PMID: 24727477 Free PMC article.
-
The Plant Mediator Complex in the Initiation of Transcription by RNA Polymerase II.Annu Rev Plant Biol. 2024 Jul;75(1):211-237. doi: 10.1146/annurev-arplant-070623-114005. Epub 2024 Jul 2. Annu Rev Plant Biol. 2024. PMID: 38277699 Review.
-
Pol II phosphorylation regulates a switch between transcriptional and splicing condensates.Nature. 2019 Aug;572(7770):543-548. doi: 10.1038/s41586-019-1464-0. Epub 2019 Aug 7. Nature. 2019. PMID: 31391587 Free PMC article.
-
Mechanisms of Mediator complex action in transcriptional activation.Cell Mol Life Sci. 2013 Aug;70(15):2743-56. doi: 10.1007/s00018-013-1265-9. Epub 2013 Jan 30. Cell Mol Life Sci. 2013. PMID: 23361037 Free PMC article. Review.
Cited by
-
Alternatively Constructed Estrogen Receptor Alpha-Driven Super-Enhancers Result in Similar Gene Expression in Breast and Endometrial Cell Lines.Int J Mol Sci. 2020 Feb 27;21(5):1630. doi: 10.3390/ijms21051630. Int J Mol Sci. 2020. PMID: 32120995 Free PMC article.
-
Allostery: Allosteric Cancer Drivers and Innovative Allosteric Drugs.J Mol Biol. 2022 Sep 15;434(17):167569. doi: 10.1016/j.jmb.2022.167569. Epub 2022 Apr 1. J Mol Biol. 2022. PMID: 35378118 Free PMC article. Review.
-
Multiple conformational selection and induced fit events take place in allosteric propagation.Biophys Chem. 2014 Feb;186:22-30. doi: 10.1016/j.bpc.2013.10.002. Epub 2013 Oct 31. Biophys Chem. 2014. PMID: 24239303 Free PMC article. Review.
-
Protein Ensembles: How Does Nature Harness Thermodynamic Fluctuations for Life? The Diverse Functional Roles of Conformational Ensembles in the Cell.Chem Rev. 2016 Jun 8;116(11):6516-51. doi: 10.1021/acs.chemrev.5b00562. Epub 2016 Jan 25. Chem Rev. 2016. PMID: 26807783 Free PMC article. Review.
-
The underappreciated role of allostery in the cellular network.Annu Rev Biophys. 2013;42:169-89. doi: 10.1146/annurev-biophys-083012-130257. Epub 2013 Feb 28. Annu Rev Biophys. 2013. PMID: 23451894 Free PMC article. Review.
References
-
- Panne D (2008) The enhanceosome. Curr. Opin. Struct. Biol 18, 236–242 - PubMed
Publication types
MeSH terms
Substances
Grants and funding
LinkOut - more resources
Full Text Sources
Miscellaneous