Physiopathology of the cochlear microcirculation
- PMID: 21875658
- PMCID: PMC3608480
- DOI: 10.1016/j.heares.2011.08.006
Physiopathology of the cochlear microcirculation
Abstract
Normal blood supply to the cochlea is critically important for establishing the endocochlear potential and sustaining production of endolymph. Abnormal cochlear microcirculation has long been considered an etiologic factor in noise-induced hearing loss, age-related hearing loss (presbycusis), sudden hearing loss or vestibular function, and Meniere's disease. Knowledge of the mechanisms underlying the pathophysiology of cochlear microcirculation is of fundamental clinical importance. A better understanding of cochlear blood flow (CoBF) will enable more effective management of hearing disorders resulting from aberrant blood flow. This review focuses on recent discoveries and findings related to the physiopathology of the cochlear microvasculature.
Published by Elsevier B.V.
Figures
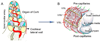
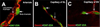
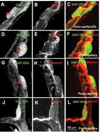
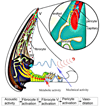
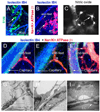
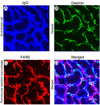
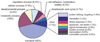
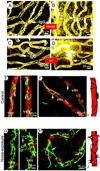
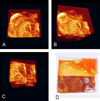
Similar articles
-
Relationship between changes in the cochlear blood flow and disorder of hearing function induced by blast injury in guinea pigs.Int J Clin Exp Pathol. 2013;6(3):375-84. Epub 2013 Feb 15. Int J Clin Exp Pathol. 2013. PMID: 23412965 Free PMC article.
-
Thin and open vessel windows for intra-vital fluorescence imaging of murine cochlear blood flow.Hear Res. 2014 Jul;313:38-46. doi: 10.1016/j.heares.2014.04.006. Epub 2014 Apr 26. Hear Res. 2014. PMID: 24780131 Free PMC article.
-
Effect of glycerol on cochlea microcirculation.Acta Otolaryngol. 1981 Nov-Dec;92(5-6):459-61. doi: 10.3109/00016488109133284. Acta Otolaryngol. 1981. PMID: 7315264
-
Mechanisms of alterations in the microcirculation of the cochlea.Ann N Y Acad Sci. 1999 Nov 28;884:226-32. doi: 10.1111/j.1749-6632.1999.tb08644.x. Ann N Y Acad Sci. 1999. PMID: 10842596 Review.
-
Animal models of inner ear vascular disturbances.Am J Otolaryngol. 1986 Mar-Apr;7(2):130-9. doi: 10.1016/s0196-0709(86)80042-4. Am J Otolaryngol. 1986. PMID: 3515984 Review.
Cited by
-
Two-photon microscopy allows imaging and characterization of cochlear microvasculature in vivo.Biomed Res Int. 2015;2015:154272. doi: 10.1155/2015/154272. Epub 2015 Mar 30. Biomed Res Int. 2015. PMID: 25883941 Free PMC article.
-
Neutrophil Extracellular Traps Affect Human Inner Ear Vascular Permeability.Int J Mol Sci. 2024 Sep 10;25(18):9766. doi: 10.3390/ijms25189766. Int J Mol Sci. 2024. PMID: 39337254 Free PMC article.
-
Audiologic profile of OSAS and simple snoring patients: the effect of chronic nocturnal intermittent hypoxia on auditory function.Eur Arch Otorhinolaryngol. 2016 Jun;273(6):1419-24. doi: 10.1007/s00405-015-3714-6. Epub 2015 Jul 12. Eur Arch Otorhinolaryngol. 2016. PMID: 26164293
-
Oxidative Stress in Obstructive Sleep Apnea Syndrome: Putative Pathways to Hearing System Impairment.Antioxidants (Basel). 2023 Jul 15;12(7):1430. doi: 10.3390/antiox12071430. Antioxidants (Basel). 2023. PMID: 37507968 Free PMC article. Review.
-
Platelet-Derived Growth Factor Subunit B Signaling Promotes Pericyte Migration in Response to Loud Sound in the Cochlear Stria Vascularis.J Assoc Res Otolaryngol. 2018 Aug;19(4):363-379. doi: 10.1007/s10162-018-0670-z. Epub 2018 Jun 4. J Assoc Res Otolaryngol. 2018. PMID: 29869048 Free PMC article.
References
-
- Abbott NJ, Ronnback L, Hansson E. Astrocyte-endothelial interactions at the blood-brain barrier. Nature reviews. 2006.;7:41–53. - PubMed
-
- Aimoni C, Bianchini C, Borin M, Ciorba A, Fellin R, Martini A, Scanelli G, Volpato S. Diabetes, cardiovascular risk factors and idiopathic sudden sensorineural hearing loss: A case-control study. Audiol Neurotol. 2010;15:111–115. - PubMed
-
- Albera R, Ferrero V, Canale A, De Siena L, Pallavicino F, Poli L. Cochlear blood flow modifications induced by anaesthetic drugs in middle ear surgery: comparison between sevoflurane and propofol. Acta oto-laryngologica. 2003;123:812–816. - PubMed
Publication types
MeSH terms
Grants and funding
- R03 DC008888-03/DC/NIDCD NIH HHS/United States
- R03 DC008888/DC/NIDCD NIH HHS/United States
- R01 DC010844-02/DC/NIDCD NIH HHS/United States
- R01 DC010844/DC/NIDCD NIH HHS/United States
- R01-NIDCD DC010844/PHS HHS/United States
- R01 DC00105/DC/NIDCD NIH HHS/United States
- F32 DC000105/DC/NIDCD NIH HHS/United States
- R01 DC010844-01A1/DC/NIDCD NIH HHS/United States
- P30-DC005983.DC 00105/DC/NIDCD NIH HHS/United States
- R03 DC008888-02S1/DC/NIDCD NIH HHS/United States
- R01 DC000105/DC/NIDCD NIH HHS/United States
- R03-DC008888/DC/NIDCD NIH HHS/United States
- R01 DC010844-03/DC/NIDCD NIH HHS/United States
- P30 DC005983/DC/NIDCD NIH HHS/United States
- DC008888S1/DC/NIDCD NIH HHS/United States
- R03 DC008888-02/DC/NIDCD NIH HHS/United States
- R03 DC008888-01A1/DC/NIDCD NIH HHS/United States
LinkOut - more resources
Full Text Sources
Medical