Boronate oxidation as a bioorthogonal reaction approach for studying the chemistry of hydrogen peroxide in living systems
- PMID: 21834525
- PMCID: PMC3178007
- DOI: 10.1021/ar200126t
Boronate oxidation as a bioorthogonal reaction approach for studying the chemistry of hydrogen peroxide in living systems
Abstract
Reactive oxygen species (ROS), such as hydrogen peroxide, are important products of oxygen metabolism that, when misregulated, can accumulate and cause oxidative stress inside cells. Accordingly, organisms have evolved molecular systems, including antioxidant metalloenzymes (such as superoxide dismutase and catalase) and an array of thiol-based redox couples, to neutralize this threat to the cell when it occurs. On the other hand, emerging evidence shows that the controlled generation of ROS, particularly H(2)O(2), is necessary to maintain cellular fitness. The identification of NADPH oxidase enzymes, which generate specific ROS and reside in virtually all cell types throughout the body, is a prime example. Indeed, a growing body of work shows that H(2)O(2) and other ROS have essential functions in healthy physiological signaling pathways. The signal-stress dichotomy of H(2)O(2) serves as a source of motivation for disentangling its beneficial from its detrimental effects on living systems. Molecular imaging of this oxygen metabolite with reaction-based probes is a powerful approach for real-time, noninvasive monitoring of H(2)O(2) chemistry in biological specimens, but two key challenges to studying H(2)O(2) in this way are chemoselectivity and bioorthogonality of probe molecules. Chemoselectivity is problematic because traditional methods for ROS detection suffer from nonspecific reactivity with other ROS. Moreover, some methods require enzymatic additives not compatible with live-cell or live-animal specimens. Additionally, bioorthogonality requires that the reactions must not compete with or disturb intrinsic cellular chemistry; this requirement is particularly critical with thiol- or metal-based couples mediating the major redox events within the cell. Chemoselective bioorthogonal reactions, such as alkyne-azide cycloadditions and related click reactions, the Staudinger-Bertozzi ligation, and the transformations used in various reaction-based molecular probes, have found widespread application in the modification, labeling, and detection of biological molecules and processes. In this Account, we summarize H(2)O(2) studies from our laboratory using the H(2)O(2)-mediated oxidation of aryl boronates to phenols as a bioorthogonal approach to detect fluxes of this important ROS in living systems. We have installed this versatile switch onto organic and inorganic scaffolds to serve as "turn-on" probes for visible and near-infrared (NIR) fluorescence, ratiometric fluorescence, time-gated lanthanide luminescence, and in vivo bioluminescence detection of H(2)O(2) in living cells and animals. Further chemical and genetic manipulations target these probes to specific organelles and other subcellular locales and can also allow them to be trapped intracellularly, enhancing their sensitivity. These novel chemical tools have revealed fundamental new biological insights into the production, localization, trafficking, and in vivo roles of H(2)O(2) in a wide variety of living systems, including immune, cancer, stem, and neural cell models.
© 2011 American Chemical Society
Figures
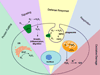
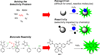
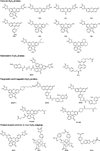
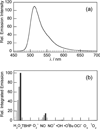
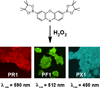
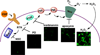
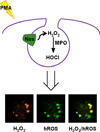
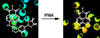
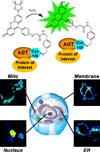
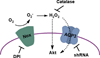
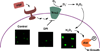
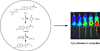
Similar articles
-
From mechanism to mouse: a tale of two bioorthogonal reactions.Acc Chem Res. 2011 Sep 20;44(9):666-76. doi: 10.1021/ar200148z. Epub 2011 Aug 15. Acc Chem Res. 2011. PMID: 21838330 Free PMC article.
-
A palette of fluorescent probes with varying emission colors for imaging hydrogen peroxide signaling in living cells.J Am Chem Soc. 2010 Apr 28;132(16):5906-15. doi: 10.1021/ja1014103. J Am Chem Soc. 2010. PMID: 20361787 Free PMC article.
-
Organelle-targetable fluorescent probes for imaging hydrogen peroxide in living cells via SNAP-Tag protein labeling.J Am Chem Soc. 2010 Mar 31;132(12):4455-65. doi: 10.1021/ja100117u. J Am Chem Soc. 2010. PMID: 20201528 Free PMC article.
-
Boronate-based fluorescent probes: imaging hydrogen peroxide in living systems.Methods Enzymol. 2013;526:19-43. doi: 10.1016/B978-0-12-405883-5.00002-8. Methods Enzymol. 2013. PMID: 23791092 Free PMC article. Review.
-
Methods for detection and measurement of hydrogen peroxide inside and outside of cells.Mol Cells. 2010 Jun;29(6):539-49. doi: 10.1007/s10059-010-0082-3. Epub 2010 Jun 4. Mol Cells. 2010. PMID: 20526816 Review.
Cited by
-
Mitochondrial hydrogen peroxide and defective cholesterol efflux prevent in vitro fertilization by cryopreserved inbred mouse sperm.Biol Reprod. 2013 Jul 25;89(1):17. doi: 10.1095/biolreprod.113.109157. Print 2013 Jul. Biol Reprod. 2013. PMID: 23740947 Free PMC article.
-
Collective activation of MRI agents via encapsulation and disease-triggered release.J Am Chem Soc. 2013 May 29;135(21):7847-50. doi: 10.1021/ja403167p. Epub 2013 May 17. J Am Chem Soc. 2013. PMID: 23672342 Free PMC article.
-
Activity-Based Sensing for Chemistry-Enabled Biology: Illuminating Principles, Probes, and Prospects for Boronate Reagents for Studying Hydrogen Peroxide.ACS Bio Med Chem Au. 2022 Dec 21;2(6):548-564. doi: 10.1021/acsbiomedchemau.2c00052. Epub 2022 Oct 11. ACS Bio Med Chem Au. 2022. PMID: 36573097 Free PMC article. Review.
-
Inorganic Chemistry Approaches to Activity-Based Sensing: From Metal Sensors to Bioorthogonal Metal Chemistry.Inorg Chem. 2019 Oct 21;58(20):13546-13560. doi: 10.1021/acs.inorgchem.9b01221. Epub 2019 Jun 7. Inorg Chem. 2019. PMID: 31185541 Free PMC article. Review.
-
In vivo targeting of hydrogen peroxide by activatable cell-penetrating peptides.J Am Chem Soc. 2014 Jan 22;136(3):874-7. doi: 10.1021/ja411547j. Epub 2014 Jan 8. J Am Chem Soc. 2014. PMID: 24377760 Free PMC article.
References
-
- Floyd RA. Oxidative damage to behavior during aging. Science. 1991;254:1597. - PubMed
-
- D’Autréaux B, Toledano MB. ROS as signaling molecules: mechanisms that generate specificty in ROS homeostasis. Nat. Rev. Mol. Cell. Biol. 2007;8:813–824. - PubMed
-
- Winterbourn CC. Reconciling the chemistry and biology of reactive oxygen species. Nat. Chem. Biol. 2008;4:278–286. - PubMed
Publication types
MeSH terms
Substances
Grants and funding
LinkOut - more resources
Full Text Sources
Other Literature Sources
Miscellaneous