A lifespan approach to neuroinflammatory and cognitive disorders: a critical role for glia
- PMID: 21822589
- PMCID: PMC3267003
- DOI: 10.1007/s11481-011-9299-y
A lifespan approach to neuroinflammatory and cognitive disorders: a critical role for glia
Abstract
Cognitive decline is a common problem of aging. Whereas multiple neural and glial mechanisms may account for these declines, microglial sensitization and/or dystrophy has emerged as a leading culprit in brain aging and dysfunction. However, glial activation is consistently observed in normal brain aging as well, independent of frank neuroinflammation or functional impairment. Such variability suggests the existence of additional vulnerability factors that can impact neuronal-glial interactions and thus overall brain and cognitive health. The goal of this review is to elucidate our working hypothesis that an individual's risk or resilience to neuroinflammatory disorders and poor cognitive aging may critically depend on their early life experience, which can change immune reactivity within the brain for the remainder of the lifespan. For instance, early-life infection in rats can profoundly disrupt memory function in young adulthood, as well as accelerate age-related cognitive decline, both of which are linked to enduring changes in glial function that occur in response to the initial infection. We discuss these findings within the context of the growing literature on the role of immune molecules and neuroimmune crosstalk in normal brain development. We highlight the intrinsic factors (e.g., chemokines, hormones) that regulate microglial development and their colonization of the embryonic and postnatal brain, and the capacity for disruption or "re-programming" of this crucial process by external events (e.g., stress, infection). An impact on glia, which in turn alters neural development, has the capacity to profoundly impact cognitive and mental health function at all stages of life.
Figures
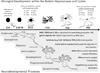
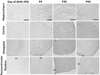
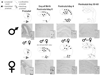
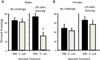
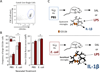
Similar articles
-
Concurrent hippocampal induction of MHC II pathway components and glial activation with advanced aging is not correlated with cognitive impairment.J Neuroinflammation. 2011 Oct 11;8:138. doi: 10.1186/1742-2094-8-138. J Neuroinflammation. 2011. PMID: 21989322 Free PMC article.
-
Age and neuroinflammation: a lifetime of psychoneuroimmune consequences.Neurol Clin. 2006 Aug;24(3):521-38. doi: 10.1016/j.ncl.2006.03.010. Neurol Clin. 2006. PMID: 16877122 Review.
-
Early-life infection is a vulnerability factor for aging-related glial alterations and cognitive decline.Neurobiol Learn Mem. 2010 Jul;94(1):57-64. doi: 10.1016/j.nlm.2010.04.001. Epub 2010 Apr 11. Neurobiol Learn Mem. 2010. PMID: 20388544 Free PMC article.
-
Cognitive and behavioral consequences of impaired immunoregulation in aging.J Neuroimmune Pharmacol. 2012 Mar;7(1):7-23. doi: 10.1007/s11481-011-9313-4. Epub 2011 Sep 20. J Neuroimmune Pharmacol. 2012. PMID: 21932047 Review.
-
Got worms? Perinatal exposure to helminths prevents persistent immune sensitization and cognitive dysfunction induced by early-life infection.Brain Behav Immun. 2016 Jan;51:14-28. doi: 10.1016/j.bbi.2015.07.006. Epub 2015 Jul 7. Brain Behav Immun. 2016. PMID: 26162711
Cited by
-
Sex differences in prefrontal cortex microglia morphology: Impact of a two-hit model of adversity throughout development.Neurosci Lett. 2020 Nov 1;738:135381. doi: 10.1016/j.neulet.2020.135381. Epub 2020 Sep 11. Neurosci Lett. 2020. PMID: 32927000 Free PMC article.
-
Glial and Neuroimmune Mechanisms as Critical Modulators of Drug Use and Abuse.Neuropsychopharmacology. 2017 Jan;42(1):156-177. doi: 10.1038/npp.2016.121. Epub 2016 Jul 11. Neuropsychopharmacology. 2017. PMID: 27402494 Free PMC article. Review.
-
Male adolescent rats display blunted cytokine responses in the CNS after acute ethanol or lipopolysaccharide exposure.Physiol Behav. 2015 Sep 1;148:131-44. doi: 10.1016/j.physbeh.2015.02.032. Epub 2015 Feb 21. Physiol Behav. 2015. PMID: 25708278 Free PMC article.
-
Prenatal stress is a vulnerability factor for altered morphology and biological activity of microglia cells.Front Cell Neurosci. 2015 Mar 12;9:82. doi: 10.3389/fncel.2015.00082. eCollection 2015. Front Cell Neurosci. 2015. PMID: 25814933 Free PMC article.
-
Impact of inflammation on developing respiratory control networks: rhythm generation, chemoreception and plasticity.Respir Physiol Neurobiol. 2020 Mar;274:103357. doi: 10.1016/j.resp.2019.103357. Epub 2019 Dec 30. Respir Physiol Neurobiol. 2020. PMID: 31899353 Free PMC article. Review.
References
-
- Abraham WC, Williams JM. Properties and mechanisms of LTP maintenance. Neuroscientist. 2003;9:463–474. - PubMed
-
- Akira S, Takeda K. Toll-like receptor signalling. Nat Rev Immunol. 2004;4:499–511. - PubMed
-
- Anderson KV, Jurgens G, Nusslein-Volhard C. Establishment of dorsal-ventral polarity in the Drosophila embryo: genetic studies on the role of the Toll gene product. Cell. 1985;42:779–789. - PubMed
-
- Araque A, Parpura V, Sanzgiri RP, Haydon PG. Tripartite synapses: glia, the unacknowledged partner. Trends Neurosci. 1999;22:208–215. - PubMed
Publication types
MeSH terms
Grants and funding
LinkOut - more resources
Full Text Sources
Medical