Retrotransposons that maintain chromosome ends
- PMID: 21821789
- PMCID: PMC3251079
- DOI: 10.1073/pnas.1100278108
Retrotransposons that maintain chromosome ends
Abstract
Reverse transcriptases have shaped genomes in many ways. A remarkable example of this shaping is found on telomeres of the genus Drosophila, where retrotransposons have a vital role in chromosome structure. Drosophila lacks telomerase; instead, three telomere-specific retrotransposons maintain chromosome ends. Repeated transpositions to chromosome ends produce long head to tail arrays of these elements. In both form and function, these arrays are analogous to the arrays of repeats added by telomerase to chromosomes in other organisms. Distantly related Drosophila exhibit this variant mechanism of telomere maintenance, which was established before the separation of extant Drosophila species. Nevertheless, the telomere-specific elements still have the hallmarks that characterize non-long terminal repeat (non-LTR) retrotransposons; they have also acquired characteristics associated with their roles at telomeres. These telomeric retrotransposons have shaped the Drosophila genome, but they have also been shaped by the genome. Here, we discuss ways in which these three telomere-specific retrotransposons have been modified for their roles in Drosophila chromosomes.
Conflict of interest statement
The authors declare no conflict of interest.
Figures
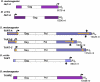
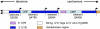
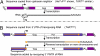
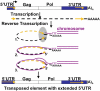
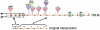
Similar articles
-
Drosophila telomeric retrotransposons derived from an ancestral element that was recruited to replace telomerase.Genome Res. 2007 Dec;17(12):1909-18. doi: 10.1101/gr.6365107. Epub 2007 Nov 7. Genome Res. 2007. PMID: 17989257 Free PMC article.
-
Transposon telomeres are widely distributed in the Drosophila genus: TART elements in the virilis group.Proc Natl Acad Sci U S A. 2003 Mar 18;100(6):3363-8. doi: 10.1073/pnas.0230353100. Epub 2003 Mar 7. Proc Natl Acad Sci U S A. 2003. PMID: 12626755 Free PMC article.
-
Two retrotransposons maintain telomeres in Drosophila.Chromosome Res. 2005;13(5):443-53. doi: 10.1007/s10577-005-0993-6. Chromosome Res. 2005. PMID: 16132810 Free PMC article. Review.
-
Adapting to life at the end of the line: How Drosophila telomeric retrotransposons cope with their job.Mob Genet Elements. 2011 Jul;1(2):128-134. doi: 10.4161/mge.1.2.16914. Epub 2011 Jul 1. Mob Genet Elements. 2011. PMID: 22016861 Free PMC article.
-
Drosophila telomeres: A variation on the telomerase theme.Fly (Austin). 2008 May-Jun;2(3):101-10. doi: 10.4161/fly.6393. Epub 2008 May 4. Fly (Austin). 2008. PMID: 18820466 Review.
Cited by
-
Identifying and correcting repeat-calling errors in nanopore sequencing of telomeres.Genome Biol. 2022 Aug 26;23(1):180. doi: 10.1186/s13059-022-02751-6. Genome Biol. 2022. PMID: 36028900 Free PMC article.
-
Untangling the web: the diverse functions of the PIWI/piRNA pathway.Mol Reprod Dev. 2013 Aug;80(8):632-64. doi: 10.1002/mrd.22195. Epub 2013 Jun 27. Mol Reprod Dev. 2013. PMID: 23712694 Free PMC article. Review.
-
Evolutionary history and classification of Micropia retroelements in Drosophilidae species.PLoS One. 2019 Oct 17;14(10):e0220539. doi: 10.1371/journal.pone.0220539. eCollection 2019. PLoS One. 2019. PMID: 31622354 Free PMC article.
-
Useful parasites: the evolutionary biology and biotechnology applications of transposable elements.J Genet. 2016 Dec;95(4):1039-1052. doi: 10.1007/s12041-016-0702-6. J Genet. 2016. PMID: 27994207 Review.
-
Evolving Linear Chromosomes and Telomeres: A C-Strand-Centric View.Trends Biochem Sci. 2018 May;43(5):314-326. doi: 10.1016/j.tibs.2018.02.008. Epub 2018 Mar 14. Trends Biochem Sci. 2018. PMID: 29550242 Free PMC article. Review.
References
Publication types
MeSH terms
Substances
Grants and funding
LinkOut - more resources
Full Text Sources
Molecular Biology Databases