Overlapping role of dynamin isoforms in synaptic vesicle endocytosis
- PMID: 21689597
- PMCID: PMC3190241
- DOI: 10.1016/j.neuron.2011.04.031
Overlapping role of dynamin isoforms in synaptic vesicle endocytosis
Abstract
The existence of neuron-specific endocytic protein isoforms raises questions about their importance for specialized neuronal functions. Dynamin, a GTPase implicated in the fission reaction of endocytosis, is encoded by three genes, two of which, dynamin 1 and 3, are highly expressed in neurons. We show that dynamin 3, thought to play a predominantly postsynaptic role, has a major presynaptic function. Although lack of dynamin 3 does not produce an overt phenotype in mice, it worsens the dynamin 1 KO phenotype, leading to perinatal lethality and a more severe defect in activity-dependent synaptic vesicle endocytosis. Thus, dynamin 1 and 3, which together account for the overwhelming majority of brain dynamin, cooperate in supporting optimal rates of synaptic vesicle endocytosis. Persistence of synaptic transmission in their absence indicates that if dynamin plays essential functions in neurons, such functions can be achieved by the very low levels of dynamin 2.
Copyright © 2011 Elsevier Inc. All rights reserved.
Figures
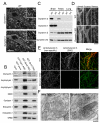
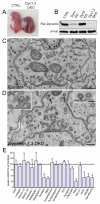
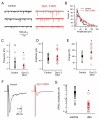
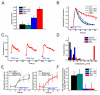
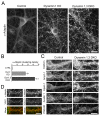
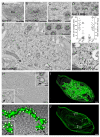
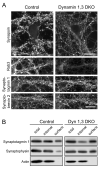
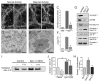
Similar articles
-
Dynamin triple knockout cells reveal off target effects of commonly used dynamin inhibitors.J Cell Sci. 2013 Nov 15;126(Pt 22):5305-12. doi: 10.1242/jcs.138578. Epub 2013 Sep 17. J Cell Sci. 2013. PMID: 24046449 Free PMC article.
-
A selective activity-dependent requirement for dynamin 1 in synaptic vesicle endocytosis.Science. 2007 Apr 27;316(5824):570-4. doi: 10.1126/science.1140621. Science. 2007. PMID: 17463283
-
Interaction of SPIN90 with dynamin I and its participation in synaptic vesicle endocytosis.J Neurosci. 2005 Oct 12;25(41):9515-23. doi: 10.1523/JNEUROSCI.1643-05.2005. J Neurosci. 2005. PMID: 16221862 Free PMC article.
-
Dynamin I phosphorylation and the control of synaptic vesicle endocytosis.Biochem Soc Symp. 2005;(72):87-97. doi: 10.1042/bss0720087. Biochem Soc Symp. 2005. PMID: 15649133 Free PMC article. Review.
-
Phosphorylation of dynamin I and synaptic-vesicle recycling.Trends Neurosci. 1994 Aug;17(8):348-53. doi: 10.1016/0166-2236(94)90179-1. Trends Neurosci. 1994. PMID: 7526507 Review.
Cited by
-
Dynamin 1- and 3-Mediated Endocytosis Is Essential for the Development of a Large Central Synapse In Vivo.J Neurosci. 2016 Jun 1;36(22):6097-115. doi: 10.1523/JNEUROSCI.3804-15.2016. J Neurosci. 2016. PMID: 27251629 Free PMC article.
-
Membrane fission by dynamin: what we know and what we need to know.EMBO J. 2016 Nov 2;35(21):2270-2284. doi: 10.15252/embj.201694613. Epub 2016 Sep 26. EMBO J. 2016. PMID: 27670760 Free PMC article. Review.
-
α-Synuclein facilitates endocytosis by elevating the steady-state levels of phosphatidylinositol 4,5-bisphosphate.J Biol Chem. 2020 Dec 25;295(52):18076-18090. doi: 10.1074/jbc.RA120.015319. Epub 2020 Oct 21. J Biol Chem. 2020. PMID: 33087443 Free PMC article.
-
Dynamin-2 function and dysfunction along the secretory pathway.Front Endocrinol (Lausanne). 2013 Sep 18;4:126. doi: 10.3389/fendo.2013.00126. Front Endocrinol (Lausanne). 2013. PMID: 24065954 Free PMC article. Review.
-
Dynamin 2 is required for GPVI signaling and platelet hemostatic function in mice.Haematologica. 2020 May;105(5):1414-1423. doi: 10.3324/haematol.2019.218644. Epub 2019 Jul 11. Haematologica. 2020. PMID: 31296575 Free PMC article.
References
-
- Anggono V, Robinson PJ. Syndapin I and endophilin I bind overlapping proline-rich regions of dynamin I: role in synaptic vesicle endocytosis. J Neurochem. 2007;102:931–943. - PubMed
-
- Aramburu J, Yaffe MB, Lopez-Rodriguez C, Cantley LC, Hogan PG, Rao A. Affinity-driven peptide selection of an NFAT inhibitor more selective than cyclosporin A. Science. 1999;285:2129–2133. - PubMed
Publication types
MeSH terms
Substances
Grants and funding
- P30 DA018343-01/DA/NIDA NIH HHS/United States
- RR-000592/RR/NCRR NIH HHS/United States
- CAPMC/ CIHR/Canada
- P41 RR000592-38/RR/NCRR NIH HHS/United States
- P30 DA018343/DA/NIDA NIH HHS/United States
- DK45735/DK/NIDDK NIH HHS/United States
- P30 DK045735/DK/NIDDK NIH HHS/United States
- P30 DK045735-10/DK/NIDDK NIH HHS/United States
- R37 NS036251-16/NS/NINDS NIH HHS/United States
- P41 RR000592/RR/NCRR NIH HHS/United States
- R37 NS036251/NS/NINDS NIH HHS/United States
- R37 NS036942/NS/NINDS NIH HHS/United States
- R01 NS036942/NS/NINDS NIH HHS/United States
- R01 NS036942-12/NS/NINDS NIH HHS/United States
- R37NS036251/NS/NINDS NIH HHS/United States
- NS36942/NS/NINDS NIH HHS/United States
LinkOut - more resources
Full Text Sources
Molecular Biology Databases
Research Materials