Synchrony makes neurons fire in sequence, and stimulus properties determine who is ahead
- PMID: 21653861
- PMCID: PMC6623348
- DOI: 10.1523/JNEUROSCI.2817-10.2011
Synchrony makes neurons fire in sequence, and stimulus properties determine who is ahead
Abstract
The synchronized activity of cortical neurons often features spike delays of several milliseconds. Usually, these delays are considered too small to play a role in cortical computations. Here, we use simultaneous recordings of spiking activity from up to 12 neurons to show that, in the cat visual cortex, the pairwise delays between neurons form a preferred order of spiking, called firing sequence. This sequence spans up to ∼ 15 ms and is referenced not to external events but to the internal cortical activity (e.g., beta/gamma oscillations). Most importantly, the preferred sequence of firing changed consistently as a function of stimulus properties. During beta/gamma oscillations, the reliability of firing sequences increased and approached that of firing rates. This suggests that, in the visual system, short-lived spatiotemporal patterns of spiking defined by consistent delays in synchronized activity occur with sufficient reliability to complement firing rates as a neuronal code.
Figures
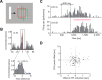
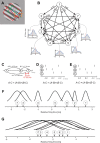
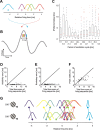
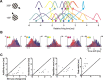
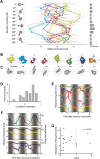
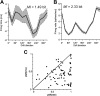
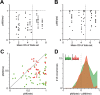
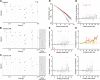
Similar articles
-
Synchrony between orientation-selective neurons is modulated during adaptation-induced plasticity in cat visual cortex.BMC Neurosci. 2008 Jul 3;9:60. doi: 10.1186/1471-2202-9-60. BMC Neurosci. 2008. PMID: 18598368 Free PMC article.
-
Synaptic origin and stimulus dependency of neuronal oscillatory activity in the primary visual cortex of the cat.J Physiol. 1997 May 1;500 ( Pt 3)(Pt 3):751-74. doi: 10.1113/jphysiol.1997.sp022056. J Physiol. 1997. PMID: 9161989 Free PMC article.
-
Stimulus-selective spiking is driven by the relative timing of synchronous excitation and disinhibition in cat striate neurons in vivo.Eur J Neurosci. 2008 Oct;28(7):1286-300. doi: 10.1111/j.1460-9568.2008.06434.x. Eur J Neurosci. 2008. PMID: 18973556
-
Pattern separation and synchronization in spiking associative memories and visual areas.Neural Netw. 2001 Jul-Sep;14(6-7):763-80. doi: 10.1016/s0893-6080(01)00084-3. Neural Netw. 2001. PMID: 11665769 Review.
-
The highly irregular firing of cortical cells is inconsistent with temporal integration of random EPSPs.J Neurosci. 1993 Jan;13(1):334-50. doi: 10.1523/JNEUROSCI.13-01-00334.1993. J Neurosci. 1993. PMID: 8423479 Free PMC article. Review.
Cited by
-
Improved measures of phase-coupling between spikes and the Local Field Potential.J Comput Neurosci. 2012 Aug;33(1):53-75. doi: 10.1007/s10827-011-0374-4. Epub 2011 Dec 21. J Comput Neurosci. 2012. PMID: 22187161 Free PMC article.
-
A new approach to solving the feature-binding problem in primate vision.Interface Focus. 2018 Aug 6;8(4):20180021. doi: 10.1098/rsfs.2018.0021. Epub 2018 Jun 15. Interface Focus. 2018. PMID: 29951198 Free PMC article.
-
Unsupervised clustering of temporal patterns in high-dimensional neuronal ensembles using a novel dissimilarity measure.PLoS Comput Biol. 2018 Jul 6;14(7):e1006283. doi: 10.1371/journal.pcbi.1006283. eCollection 2018 Jul. PLoS Comput Biol. 2018. PMID: 29979681 Free PMC article.
-
STDP Allows Close-to-Optimal Spatiotemporal Spike Pattern Detection by Single Coincidence Detector Neurons.Neuroscience. 2018 Oct 1;389:133-140. doi: 10.1016/j.neuroscience.2017.06.032. Epub 2017 Jun 29. Neuroscience. 2018. PMID: 28668487 Free PMC article.
-
Task-dependent changes in cross-level coupling between single neurons and oscillatory activity in multiscale networks.PLoS Comput Biol. 2012;8(12):e1002809. doi: 10.1371/journal.pcbi.1002809. Epub 2012 Dec 20. PLoS Comput Biol. 2012. PMID: 23284276 Free PMC article.
References
-
- Ahissar E, Arieli A. Figuring space by time. Neuron. 2001;32:185–201. - PubMed
-
- Alonso JM, Martinez LM. Functional connectivity between simple cells and complex cells in cat striate cortex. Nat Neurosci. 1998;1:395–403. - PubMed
-
- Azouz R, Gray CM. Adaptive coincidence detection and dynamic gain control in visual cortical neurons in vivo. Neuron. 2003;37:513–523. - PubMed
-
- Berényi A, Benedek G, Nagy A. Double sliding-window technique: a new method to calculate the neuronal response onset latency. Brain Res. 2007;1178:141–148. - PubMed
Publication types
MeSH terms
LinkOut - more resources
Full Text Sources
Miscellaneous