Retinoic acid-inducible gene I-inducible miR-23b inhibits infections by minor group rhinoviruses through down-regulation of the very low density lipoprotein receptor
- PMID: 21642441
- PMCID: PMC3138319
- DOI: 10.1074/jbc.M111.229856
Retinoic acid-inducible gene I-inducible miR-23b inhibits infections by minor group rhinoviruses through down-regulation of the very low density lipoprotein receptor
Abstract
In mammals, viral infections are detected by innate immune receptors, including Toll-like receptor and retinoic acid inducible gene I (RIG-I)-like receptor (RLR), which activate the type I interferon (IFN) system. IFN essentially activates genes encoding antiviral proteins that inhibit various steps of viral replication as well as facilitate the subsequent activation of acquired immune responses. In this study, we investigated the expression of non-coding RNA upon viral infection or RLR activation. Using a microarray, we identified several microRNAs (miRNA) specifically induced to express by RLR signaling. As suggested by Bioinformatics (miRBase Target Data base), one of the RLR-inducible miRNAs, miR-23b, actually knocked down the expression of very low density lipoprotein receptor (VLDLR) and LDLR-related protein 5 (LRP5). Transfection of miR-23b specifically inhibited infection of rhinovirus 1B (RV1B), which utilizes the low density lipoprotein receptor (LDLR) family for viral entry. Conversely, introduction of anti-miRNA-23b enhanced the viral yield. Knockdown experiments using small interfering RNA (siRNA) revealed that VLDLR, but not LRP5, is critical for an efficient infection by RV1B. Furthermore, experiments with the transfection of infectious viral RNA revealed that miR-23b did not affect post-entry viral replication. Our results strongly suggest that RIG-I signaling results in the inhibitions of infections of RV1B through the miR-23b-mediated down-regulation of its receptor VLDLR.
Figures
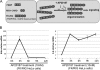
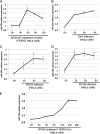
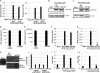
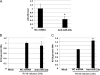
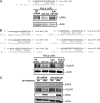
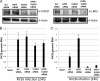
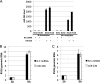
Similar articles
-
TLR3 and MDA5 signalling, although not expression, is impaired in asthmatic epithelial cells in response to rhinovirus infection.Clin Exp Allergy. 2014 Jan;44(1):91-101. doi: 10.1111/cea.12218. Clin Exp Allergy. 2014. PMID: 24131248
-
5'-triphosphate-siRNA activates RIG-I-dependent type I interferon production and enhances inhibition of hepatitis B virus replication in HepG2.2.15 cells.Eur J Pharmacol. 2013 Dec 5;721(1-3):86-95. doi: 10.1016/j.ejphar.2013.09.050. Epub 2013 Oct 5. Eur J Pharmacol. 2013. PMID: 24099962
-
Retinoic Acid Inducible Gene 1 Protein (RIG1)-Like Receptor Pathway Is Required for Efficient Nuclear Reprogramming.Stem Cells. 2017 May;35(5):1197-1207. doi: 10.1002/stem.2607. Epub 2017 Mar 27. Stem Cells. 2017. PMID: 28276156 Free PMC article.
-
Regulation of RLR-mediated innate immune signaling--it is all about keeping the balance.Eur J Cell Biol. 2012 Jan;91(1):36-47. doi: 10.1016/j.ejcb.2011.01.011. Epub 2011 Apr 9. Eur J Cell Biol. 2012. PMID: 21481967 Review.
-
[Role of the RIG-I-like receptors in antiviral response].Postepy Hig Med Dosw (Online). 2014 Jan 2;68:541-56. doi: 10.5604/17322693.1102281. Postepy Hig Med Dosw (Online). 2014. PMID: 24864105 Review. Polish.
Cited by
-
Identification of host miRNAs that may limit human rhinovirus replication.World J Biol Chem. 2014 Nov 26;5(4):437-56. doi: 10.4331/wjbc.v5.i4.437. World J Biol Chem. 2014. PMID: 25426267 Free PMC article.
-
How do deer respiratory epithelial cells weather the initial storm of SARS-CoV-2 WA1/2020 strain?Microbiol Spectr. 2024 Feb 6;12(2):e0252423. doi: 10.1128/spectrum.02524-23. Epub 2024 Jan 8. Microbiol Spectr. 2024. PMID: 38189329 Free PMC article.
-
The role of microRNAs in enteroviral infections.Braz J Infect Dis. 2015 Sep-Oct;19(5):510-6. doi: 10.1016/j.bjid.2015.06.011. Epub 2015 Sep 3. Braz J Infect Dis. 2015. PMID: 26342975 Free PMC article. Review.
-
MicroRNA-23b Promotes Avian Leukosis Virus Subgroup J (ALV-J) Replication by Targeting IRF1.Sci Rep. 2015 May 18;5:10294. doi: 10.1038/srep10294. Sci Rep. 2015. PMID: 25980475 Free PMC article.
-
KSHV-Encoded MicroRNAs: Lessons for Viral Cancer Pathogenesis and Emerging Concepts.Int J Cell Biol. 2012;2012:603961. doi: 10.1155/2012/603961. Epub 2012 Feb 19. Int J Cell Biol. 2012. PMID: 22505930 Free PMC article.
References
-
- Vaucheret H., Béclin C., Fagard M. (2001) J. Cell Sci. 114, 3083–3091 - PubMed
-
- Takaoka A., Yanai H. (2006) Cell. Microbiol. 8, 907–922 - PubMed
-
- Baulcombe D. (2004) Nature 431, 356–363 - PubMed
-
- Akira S., Takeda K. (2004) Nat. Rev. Immunol. 4, 499–511 - PubMed
-
- Yoneyama M., Kikuchi M., Matsumoto K., Imaizumi T., Miyagishi M., Taira K., Foy E., Loo Y. M., Gale M., Jr., Akira S., Yonehara S., Kato A., Fujita T. (2005) J. Immunol. 175, 2851–2858 - PubMed
MeSH terms
Substances
Grants and funding
LinkOut - more resources
Full Text Sources
Research Materials