VPS29 is not an active metallo-phosphatase but is a rigid scaffold required for retromer interaction with accessory proteins
- PMID: 21629666
- PMCID: PMC3101248
- DOI: 10.1371/journal.pone.0020420
VPS29 is not an active metallo-phosphatase but is a rigid scaffold required for retromer interaction with accessory proteins
Abstract
VPS29 is a key component of the cargo-binding core complex of retromer, a protein assembly with diverse roles in transport of receptors within the endosomal system. VPS29 has a fold related to metal-binding phosphatases and mediates interactions between retromer and other regulatory proteins. In this study we examine the functional interactions of mammalian VPS29, using X-ray crystallography and NMR spectroscopy. We find that although VPS29 can coordinate metal ions Mn(2+) and Zn(2+) in both the putative active site and at other locations, the affinity for metals is low, and lack of activity in phosphatase assays using a putative peptide substrate support the conclusion that VPS29 is not a functional metalloenzyme. There is evidence that structural elements of VPS29 critical for binding the retromer subunit VPS35 may undergo both metal-dependent and independent conformational changes regulating complex formation, however studies using ITC and NMR residual dipolar coupling (RDC) measurements show that this is not the case. Finally, NMR chemical shift mapping indicates that VPS29 is able to associate with SNX1 via a conserved hydrophobic surface, but with a low affinity that suggests additional interactions will be required to stabilise the complex in vivo. Our conclusion is that VPS29 is a metal ion-independent, rigid scaffolding domain, which is essential but not sufficient for incorporation of retromer into functional endosomal transport assemblies.
Conflict of interest statement
Figures
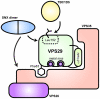
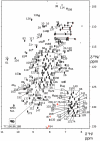
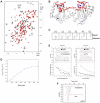
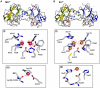
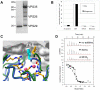
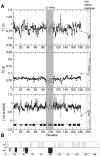
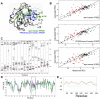
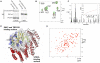
Similar articles
-
Interchangeable but essential functions of SNX1 and SNX2 in the association of retromer with endosomes and the trafficking of mannose 6-phosphate receptors.Mol Cell Biol. 2007 Feb;27(3):1112-24. doi: 10.1128/MCB.00156-06. Epub 2006 Nov 13. Mol Cell Biol. 2007. PMID: 17101778 Free PMC article.
-
Assembly and solution structure of the core retromer protein complex.Traffic. 2011 Jan;12(1):56-71. doi: 10.1111/j.1600-0854.2010.01124.x. Epub 2010 Oct 15. Traffic. 2011. PMID: 20875039
-
Functional architecture of the retromer cargo-recognition complex.Nature. 2007 Oct 25;449(7165):1063-7. doi: 10.1038/nature06216. Epub 2007 Sep 23. Nature. 2007. PMID: 17891154 Free PMC article.
-
Retromer.Curr Opin Cell Biol. 2008 Aug;20(4):427-36. doi: 10.1016/j.ceb.2008.03.009. Epub 2008 May 9. Curr Opin Cell Biol. 2008. PMID: 18472259 Free PMC article. Review.
-
Retromer: Structure, function, and roles in mammalian disease.Eur J Cell Biol. 2015 Nov;94(11):513-21. doi: 10.1016/j.ejcb.2015.07.002. Epub 2015 Jul 17. Eur J Cell Biol. 2015. PMID: 26220253 Review.
Cited by
-
Retromer Endosome Exit Domains Serve Multiple Trafficking Destinations and Regulate Local G Protein Activation by GPCRs.Curr Biol. 2016 Dec 5;26(23):3129-3142. doi: 10.1016/j.cub.2016.09.052. Epub 2016 Nov 10. Curr Biol. 2016. PMID: 27839977 Free PMC article.
-
Receptor Recycling by Retromer.Mol Cell Biol. 2023;43(7):317-334. doi: 10.1080/10985549.2023.2222053. Epub 2023 Jun 23. Mol Cell Biol. 2023. PMID: 37350516 Free PMC article. Review.
-
Emerging Role of Retromer in Modulating Pathogen Growth.Trends Microbiol. 2018 Sep;26(9):769-780. doi: 10.1016/j.tim.2018.04.001. Epub 2018 Apr 24. Trends Microbiol. 2018. PMID: 29703496 Free PMC article. Review.
-
VARP is recruited on to endosomes by direct interaction with retromer, where together they function in export to the cell surface.Dev Cell. 2014 Jun 9;29(5):591-606. doi: 10.1016/j.devcel.2014.04.010. Epub 2014 May 22. Dev Cell. 2014. PMID: 24856514 Free PMC article.
-
Identification of molecular heterogeneity in SNX27-retromer-mediated endosome-to-plasma-membrane recycling.J Cell Sci. 2014 Nov 15;127(Pt 22):4940-53. doi: 10.1242/jcs.156299. Epub 2014 Oct 2. J Cell Sci. 2014. PMID: 25278552 Free PMC article.
References
Publication types
MeSH terms
Substances
Grants and funding
LinkOut - more resources
Full Text Sources
Molecular Biology Databases
Miscellaneous