The DISABLED protein functions in CLATHRIN-mediated synaptic vesicle endocytosis and exoendocytic coupling at the active zone
- PMID: 21606364
- PMCID: PMC3121831
- DOI: 10.1073/pnas.1102231108
The DISABLED protein functions in CLATHRIN-mediated synaptic vesicle endocytosis and exoendocytic coupling at the active zone
Abstract
Members of the DISABLED (DAB) family of proteins are known to play a conserved role in endocytic trafficking of cell surface receptors by functioning as monomeric CLATHRIN-associated sorting proteins that recruit cargo proteins into endocytic vesicles. Here, we report a Drosophila disabled mutant revealing a novel role for DAB proteins in chemical synaptic transmission. This mutant exhibits impaired synaptic function, including a rapid activity-dependent reduction in neurotransmitter release and disruption of synaptic vesicle endocytosis. In presynaptic boutons, Drosophila DAB and CLATHRIN were highly colocalized within two distinct classes of puncta, including relatively dim puncta that were located at active zones and may reflect endocytic mechanisms operating at neurotransmitter release sites. Finally, broader analysis of endocytic proteins, including DYNAMIN, supported a general role for CLATHRIN-mediated endocytic mechanisms in rapid clearance of neurotransmitter release sites for subsequent vesicle priming and refilling of the release-ready vesicle pool.
Conflict of interest statement
The authors declare no conflict of interest.
Figures
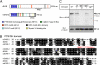
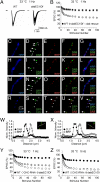
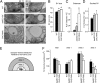
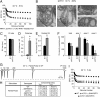
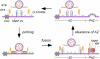
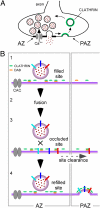
Similar articles
-
Interaction of stoned and synaptotagmin in synaptic vesicle endocytosis.J Neurosci. 2001 Feb 15;21(4):1218-27. doi: 10.1523/JNEUROSCI.21-04-01218.2001. J Neurosci. 2001. PMID: 11160392 Free PMC article.
-
The synaptic vesicle cluster: a source of endocytic proteins during neurotransmitter release.Neuroscience. 2009 Jan 12;158(1):204-10. doi: 10.1016/j.neuroscience.2008.03.035. Epub 2008 Mar 26. Neuroscience. 2009. PMID: 18440714 Review.
-
A conserved clathrin assembly motif essential for synaptic vesicle endocytosis.J Neurosci. 2000 Dec 1;20(23):8667-76. doi: 10.1523/JNEUROSCI.20-23-08667.2000. J Neurosci. 2000. PMID: 11102472 Free PMC article.
-
Formation of an endophilin-Ca2+ channel complex is critical for clathrin-mediated synaptic vesicle endocytosis.Cell. 2003 Oct 3;115(1):37-48. doi: 10.1016/s0092-8674(03)00726-8. Cell. 2003. PMID: 14532001
-
Clathrin-mediated endocytosis at the synaptic terminal: bridging the gap between physiology and molecules.Traffic. 2010 Dec;11(12):1489-97. doi: 10.1111/j.1600-0854.2010.01104.x. Traffic. 2010. PMID: 20633242 Free PMC article. Review.
Cited by
-
Lipophorin receptors regulate mushroom body development and complex behaviors in Drosophila.BMC Biol. 2022 Sep 7;20(1):198. doi: 10.1186/s12915-022-01393-1. BMC Biol. 2022. PMID: 36071487 Free PMC article.
-
Active zone proteins are transported via distinct mechanisms regulated by Par-1 kinase.PLoS Genet. 2017 Feb 21;13(2):e1006621. doi: 10.1371/journal.pgen.1006621. eCollection 2017 Feb. PLoS Genet. 2017. PMID: 28222093 Free PMC article.
-
Molecular mechanisms of COMPLEXIN fusion clamp function in synaptic exocytosis revealed in a new Drosophila mutant.Mol Cell Neurosci. 2013 Sep;56:244-54. doi: 10.1016/j.mcn.2013.06.002. Epub 2013 Jun 11. Mol Cell Neurosci. 2013. PMID: 23769723 Free PMC article.
-
Adapting for endocytosis: roles for endocytic sorting adaptors in directing neural development.Front Cell Neurosci. 2015 Apr 8;9:119. doi: 10.3389/fncel.2015.00119. eCollection 2015. Front Cell Neurosci. 2015. PMID: 25904845 Free PMC article. Review.
-
A Distinct Perisynaptic Glial Cell Type Forms Tripartite Neuromuscular Synapses in the Drosophila Adult.PLoS One. 2015 Jun 8;10(6):e0129957. doi: 10.1371/journal.pone.0129957. eCollection 2015. PLoS One. 2015. PMID: 26053860 Free PMC article.
References
-
- Jung N, Haucke V. Clathrin-mediated endocytosis at synapses. Traffic. 2007;8:1129–1136. - PubMed
-
- Shupliakov O, Brodin L. Recent insights into the building and cycling of synaptic vesicles. Exp Cell Res. 2010;316:1344–1350. - PubMed
-
- Dittman J, Ryan TA. Molecular circuitry of endocytosis at nerve terminals. Annu Rev Cell Dev Biol. 2009;25:133–160. - PubMed
-
- Gertler FB, Bennett RL, Clark MJ, Hoffmann FM. Drosophila abl tyrosine kinase in embryonic CNS axons: A role in axonogenesis is revealed through dosage-sensitive interactions with disabled. Cell. 1989;58:103–113. - PubMed
Publication types
MeSH terms
Substances
Grants and funding
LinkOut - more resources
Full Text Sources
Molecular Biology Databases
Miscellaneous