Adapter protein SH2B1beta binds filamin A to regulate prolactin-dependent cytoskeletal reorganization and cell motility
- PMID: 21566085
- PMCID: PMC3125092
- DOI: 10.1210/me.2011-0056
Adapter protein SH2B1beta binds filamin A to regulate prolactin-dependent cytoskeletal reorganization and cell motility
Abstract
Prolactin (PRL) regulates cytoskeletal rearrangement and cell motility. PRL-activated Janus tyrosine kinase 2 (JAK2) phosphorylates the p21-activated serine-threonine kinase (PAK)1 and the Src homology 2 (SH2) domain-containing adapter protein SH2B1β. SH2B1β is an actin-binding protein that cross-links actin filaments, whereas PAK1 regulates the actin cytoskeleton by different mechanisms, including direct phosphorylation of the actin-binding protein filamin A (FLNa). Here, we have used a FLNa-deficient human melanoma cell line (M2) and its derivative line (A7) that stably expresses FLNa to demonstrate that SH2B1β and FLNa are required for maximal PRL-dependent cell ruffling. We have found that in addition to two actin-binding domains, SH2B1β has a FLNa-binding domain (amino acids 200-260) that binds directly to repeats 17-23 of FLNa. The SH2B1β-FLNa interaction participates in PRL-dependent actin rearrangement. We also show that phosphorylation of the three tyrosines of PAK1 by JAK2, as well as the presence of FLNa, play a role in PRL-dependent cell ruffling. Finally, we show that the actin- and FLNa-binding-deficient mutant of SH2B1β (SH2B1β 3Δ) abolished PRL-dependent ruffling and PRL-dependent cell migration when expressed along with PAK1 Y3F (JAK2 tyrosyl-phosphorylation-deficient mutant). Together, these data provide insight into a novel mechanism of PRL-stimulated regulation of the actin cytoskeleton and cell motility via JAK2 signaling through FLNa, PAK1, and SH2B1β. We propose a model for PRL-dependent regulation of the actin cytoskeleton that integrates our findings with previous studies.
Figures
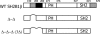
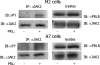
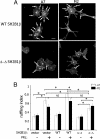
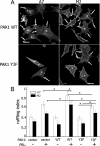
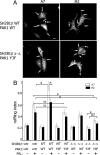
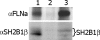
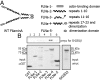
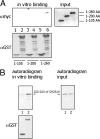
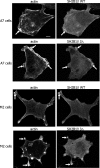
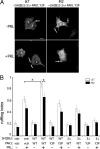
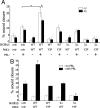
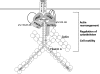
Similar articles
-
Tyrosyl phosphorylated PAK1 regulates breast cancer cell motility in response to prolactin through filamin A.Mol Endocrinol. 2013 Mar;27(3):455-65. doi: 10.1210/me.2012-1291. Epub 2013 Jan 22. Mol Endocrinol. 2013. PMID: 23340249 Free PMC article.
-
Adapter protein SH2B1beta cross-links actin filaments and regulates actin cytoskeleton.Mol Endocrinol. 2009 Jul;23(7):1065-76. doi: 10.1210/me.2008-0428. Epub 2009 Apr 2. Mol Endocrinol. 2009. PMID: 19342444 Free PMC article.
-
Filamin is essential in actin cytoskeletal assembly mediated by p21-activated kinase 1.Nat Cell Biol. 2002 Sep;4(9):681-90. doi: 10.1038/ncb838. Nat Cell Biol. 2002. PMID: 12198493
-
Tyrosyl phosphorylated serine-threonine kinase PAK1 is a novel regulator of prolactin-dependent breast cancer cell motility and invasion.Adv Exp Med Biol. 2015;846:97-137. doi: 10.1007/978-3-319-12114-7_5. Adv Exp Med Biol. 2015. PMID: 25472536 Free PMC article. Review.
-
The filamins: organizers of cell structure and function.Cell Adh Migr. 2011 Mar-Apr;5(2):160-9. doi: 10.4161/cam.5.2.14401. Epub 2011 Mar 1. Cell Adh Migr. 2011. PMID: 21169733 Free PMC article. Review.
Cited by
-
ENC1 Facilitates Colorectal Carcinoma Tumorigenesis and Metastasis via JAK2/STAT5/AKT Axis-Mediated Epithelial Mesenchymal Transition and Stemness.Front Cell Dev Biol. 2021 Mar 16;9:616887. doi: 10.3389/fcell.2021.616887. eCollection 2021. Front Cell Dev Biol. 2021. PMID: 33816464 Free PMC article.
-
Hepatic SH2B1 and SH2B2 regulate liver lipid metabolism and VLDL secretion in mice.PLoS One. 2013 Dec 17;8(12):e83269. doi: 10.1371/journal.pone.0083269. eCollection 2013. PLoS One. 2013. PMID: 24358267 Free PMC article.
-
SH2B1 promotes epithelial-mesenchymal transition through the IRS1/β-catenin signaling axis in lung adenocarcinoma.Mol Carcinog. 2018 May;57(5):640-652. doi: 10.1002/mc.22788. Epub 2018 Feb 20. Mol Carcinog. 2018. PMID: 29380446 Free PMC article.
-
Growth hormone-releasing hormone receptor antagonists inhibit human gastric cancer through downregulation of PAK1-STAT3/NF-κB signaling.Proc Natl Acad Sci U S A. 2016 Dec 20;113(51):14745-14750. doi: 10.1073/pnas.1618582114. Epub 2016 Dec 7. Proc Natl Acad Sci U S A. 2016. PMID: 27930339 Free PMC article.
-
Phosphorylation of the adaptor protein SH2B1β regulates its ability to enhance growth hormone-dependent macrophage motility.J Cell Sci. 2013 Apr 15;126(Pt 8):1733-43. doi: 10.1242/jcs.113050. Epub 2013 Feb 26. J Cell Sci. 2013. PMID: 23444381 Free PMC article.
References
-
- Bole-Feysot C, Goffin V, Edery M, Binart N, Kelly PA. 1998. Prolactin (PRL) and its receptor: actions, signal transduction pathways and phenotypes observed in PRL receptor knockout mice. Endocr Rev 19:225–268 - PubMed
-
- Horseman ND. 1999. Prolactin and mammary gland development. J Mammary Gland Biol Neoplasia 4:79–88 - PubMed
-
- Goffin V, Bernichtein S, Touraine P, Kelly PA. 2005. Development and potential clinical uses of human prolactin receptor antagonists. Endocr Rev 26:400–422 - PubMed
-
- Bernichtein S, Touraine P, Goffin V. 2010. New concepts in prolactin biology. J Endocrinol 206:1–11 - PubMed
Publication types
MeSH terms
Substances
Grants and funding
LinkOut - more resources
Full Text Sources
Research Materials
Miscellaneous