Biomimetic micro∕nanostructured functional surfaces for microfluidic and tissue engineering applications
- PMID: 21522501
- PMCID: PMC3082348
- DOI: 10.1063/1.3553235
Biomimetic micro∕nanostructured functional surfaces for microfluidic and tissue engineering applications
Abstract
This paper reviews our work on the application of ultrafast pulsed laser micro∕nanoprocessing for the three-dimensional (3D) biomimetic modification of materials surfaces. It is shown that the artificial surfaces obtained by femtosecond-laser processing of Si in reactive gas atmosphere exhibit roughness at both micro- and nanoscales that mimics the hierarchical morphology of natural surfaces. Along with the spatial control of the topology, defining surface chemistry provides materials exhibiting notable wetting characteristics which are potentially useful for open microfluidic applications. Depending on the functional coating deposited on the laser patterned 3D structures, we can achieve artificial surfaces that are (a) of extremely low surface energy, thus water-repellent and self-cleaned, and (b) responsive, i.e., showing the ability to change their surface energy in response to different external stimuli such as light, electric field, and pH. Moreover, the behavior of different kinds of cells cultured on laser engineered substrates of various wettabilities was investigated. Experiments showed that it is possible to preferentially tune cell adhesion and growth through choosing proper combinations of surface topography and chemistry. It is concluded that the laser textured 3D micro∕nano-Si surfaces with controllability of roughness ratio and surface chemistry can advantageously serve as a novel means to elucidate the 3D cell-scaffold interactions for tissue engineering applications.
Figures
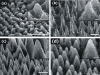
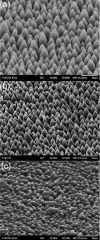
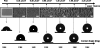
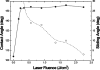
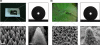
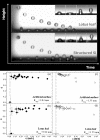
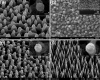
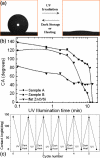
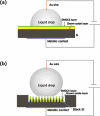
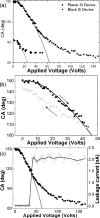
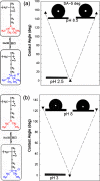
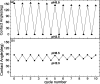
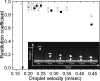
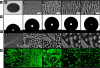
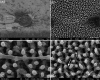
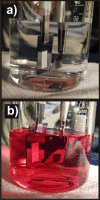
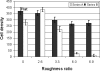
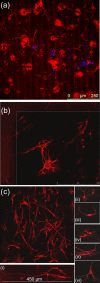
Similar articles
-
Depressing time: Waiting, melancholia, and the psychoanalytic practice of care.In: Kirtsoglou E, Simpson B, editors. The Time of Anthropology: Studies of Contemporary Chronopolitics. Abingdon: Routledge; 2020. Chapter 5. In: Kirtsoglou E, Simpson B, editors. The Time of Anthropology: Studies of Contemporary Chronopolitics. Abingdon: Routledge; 2020. Chapter 5. PMID: 36137063 Free Books & Documents. Review.
-
Enabling Systemic Identification and Functionality Profiling for Cdc42 Homeostatic Modulators.bioRxiv [Preprint]. 2024 Jan 8:2024.01.05.574351. doi: 10.1101/2024.01.05.574351. bioRxiv. 2024. Update in: Commun Chem. 2024 Nov 19;7(1):271. doi: 10.1038/s42004-024-01352-7 PMID: 38260445 Free PMC article. Updated. Preprint.
-
Defining the optimum strategy for identifying adults and children with coeliac disease: systematic review and economic modelling.Health Technol Assess. 2022 Oct;26(44):1-310. doi: 10.3310/ZUCE8371. Health Technol Assess. 2022. PMID: 36321689 Free PMC article.
-
Far Posterior Approach for Rib Fracture Fixation: Surgical Technique and Tips.JBJS Essent Surg Tech. 2024 Dec 6;14(4):e23.00094. doi: 10.2106/JBJS.ST.23.00094. eCollection 2024 Oct-Dec. JBJS Essent Surg Tech. 2024. PMID: 39650795 Free PMC article.
-
A consideration of the role of gas/particle partitioning in the deposition of nicotine and other tobacco smoke compounds in the respiratory tract.Chem Res Toxicol. 2001 Nov;14(11):1465-81. doi: 10.1021/tx0100901. Chem Res Toxicol. 2001. PMID: 11712903 Review.
Cited by
-
Preface to Special Topic: Biological microfluidics in tissue engineering and regenerative medicine.Biomicrofluidics. 2011 Mar 30;5(1):13301. doi: 10.1063/1.3571478. Biomicrofluidics. 2011. PMID: 21522490 Free PMC article.
-
Femtosecond-Laser-Produced Underwater "Superpolymphobic" Nanorippled Surfaces: Repelling Liquid Polymers in Water for Applications of Controlling Polymer Shape and Adhesion.ACS Appl Nano Mater. 2019 Nov 22;2(11):7362-7371. doi: 10.1021/acsanm.9b01869. Epub 2019 Oct 25. ACS Appl Nano Mater. 2019. PMID: 31788665 Free PMC article.
-
Enhanced pool-boiling heat transfer and critical heat flux on femtosecond laser processed stainless steel surfaces.Int J Heat Mass Transf. 2015 Mar;82:109-116. doi: 10.1016/j.ijheatmasstransfer.2014.11.023. Epub 2014 Nov 28. Int J Heat Mass Transf. 2015. PMID: 30449897 Free PMC article.
-
Ionisation processes and laser induced periodic surface structures in dielectrics with mid-infrared femtosecond laser pulses.Sci Rep. 2020 May 26;10(1):8675. doi: 10.1038/s41598-020-65613-w. Sci Rep. 2020. PMID: 32457397 Free PMC article.
-
Engineering Cell Adhesion and Orientation via Ultrafast Laser Fabricated Microstructured Substrates.Int J Mol Sci. 2018 Jul 14;19(7):2053. doi: 10.3390/ijms19072053. Int J Mol Sci. 2018. PMID: 30011926 Free PMC article.
References
-
- Stratakis E. and Zorba V., in Biomimetic and Bioinspired Nanomaterials, Nanomaterials for the Life Sciences Vol. 7, edited by Kumar C. S. S. R. (Wiley, Weinheim, 2010), Chap. 11.
-
- Xia F. and Jiang L., Adv. Mater. (Weinheim, Ger.) ADVMEW 20, 2842 (2008).10.1002/adma.200800836 - DOI
-
- Shiu J. -Y., Kuo C. -W., Chen P., and Mou C. -Y., Chem. Mater. CMATEX 16, 561 (2004).10.1021/cm034696h - DOI
-
- Bartlett P. N., Baumberg J. J., Birkin P. R., Ghanem M. A., and Netti M. C., Chem. Mater. CMATEX 14, 2199 (2002).10.1021/cm011272j - DOI
-
- Woodward I., Schofield W. C. E., Roucoules V., and Badyal J. P. S., Langmuir LANGD5 19, 3432 (2003).10.1021/la020427e - DOI
LinkOut - more resources
Full Text Sources
Other Literature Sources