The tumor necrosis factor family member LIGHT is a target for asthmatic airway remodeling
- PMID: 21499267
- PMCID: PMC3097134
- DOI: 10.1038/nm.2356
The tumor necrosis factor family member LIGHT is a target for asthmatic airway remodeling
Abstract
Individuals with chronic asthma show a progressive decline in lung function that is thought to be due to structural remodeling of the airways characterized by subepithelial fibrosis and smooth muscle hyperplasia. Here we show that the tumor necrosis factor (TNF) family member LIGHT is expressed on lung inflammatory cells after allergen exposure. Pharmacological inhibition of LIGHT using a fusion protein between the IgG Fc domain and lymphotoxin β receptor (LTβR) reduces lung fibrosis, smooth muscle hyperplasia and airway hyperresponsiveness in mouse models of chronic asthma, despite having little effect on airway eosinophilia. LIGHT-deficient mice also show a similar impairment in fibrosis and smooth muscle accumulation. Blockade of LIGHT suppresses expression of lung transforming growth factor-β (TGF-β) and interleukin-13 (IL-13), cytokines implicated in remodeling in humans, whereas exogenous administration of LIGHT to the airways induces fibrosis and smooth muscle hyperplasia, Thus, LIGHT may be targeted to prevent asthma-related airway remodeling.
Figures
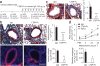
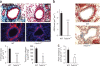
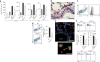
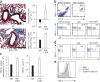
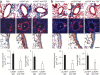
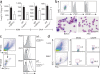
Comment in
-
Shedding LIGHT on severe asthma.Nat Med. 2011 May;17(5):547-8. doi: 10.1038/nm0511-547. Nat Med. 2011. PMID: 21546971 Free PMC article.
-
Inhibiting or blocking LIGHT, a TNF superfamily member, for treating airway remodeling.Expert Rev Respir Med. 2011 Oct;5(5):623-5. doi: 10.1586/ers.11.65. Expert Rev Respir Med. 2011. PMID: 21955232 No abstract available.
Similar articles
-
Tumor necrosis factor family member LIGHT acts with IL-1β and TGF-β to promote airway remodeling during rhinovirus infection.Allergy. 2018 Jul;73(7):1415-1424. doi: 10.1111/all.13390. Epub 2018 Mar 1. Allergy. 2018. PMID: 29315623 Free PMC article.
-
Lymphotoxin beta receptor signaling directly controls airway smooth muscle deregulation and asthmatic lung dysfunction.J Allergy Clin Immunol. 2023 Apr;151(4):976-990.e5. doi: 10.1016/j.jaci.2022.11.016. Epub 2022 Dec 5. J Allergy Clin Immunol. 2023. PMID: 36473503 Free PMC article.
-
Role of transforming growth factor-β in airway remodeling in asthma.Am J Respir Cell Mol Biol. 2011 Feb;44(2):127-33. doi: 10.1165/rcmb.2010-0027TR. Epub 2010 Jun 4. Am J Respir Cell Mol Biol. 2011. PMID: 20525803 Review.
-
TNFSF14 (LIGHT) Exhibits Inflammatory Activities in Lung Fibroblasts Complementary to IL-13 and TGF-β.Front Immunol. 2018 Mar 19;9:576. doi: 10.3389/fimmu.2018.00576. eCollection 2018. Front Immunol. 2018. PMID: 29616048 Free PMC article.
-
Structural aspects of airway remodeling in asthma.Curr Allergy Asthma Rep. 2008 Nov;8(6):540-7. doi: 10.1007/s11882-008-0098-3. Curr Allergy Asthma Rep. 2008. PMID: 18940147 Review.
Cited by
-
Altered Dermal Fibroblasts in Systemic Sclerosis Display Podoplanin and CD90.Am J Pathol. 2016 Oct;186(10):2650-64. doi: 10.1016/j.ajpath.2016.06.020. Epub 2016 Aug 23. Am J Pathol. 2016. PMID: 27565038 Free PMC article.
-
PIP-seq identifies novel heterogeneous lung innate lymphocyte population activation after combustion product exposure.Sci Rep. 2024 Aug 30;14(1):20167. doi: 10.1038/s41598-024-70880-y. Sci Rep. 2024. PMID: 39215111 Free PMC article.
-
How Mouse Macrophages Sense What Is Going On.Front Immunol. 2016 Jun 2;7:204. doi: 10.3389/fimmu.2016.00204. eCollection 2016. Front Immunol. 2016. PMID: 27313577 Free PMC article. Review.
-
The tumor necrosis factor family member TNFSF14 (LIGHT) is required for resolution of intestinal inflammation in mice.Gastroenterology. 2014 Jun;146(7):1752-62.e4. doi: 10.1053/j.gastro.2014.02.010. Epub 2014 Feb 19. Gastroenterology. 2014. PMID: 24560868 Free PMC article.
-
Virus-like particles presenting interleukin-33 molecules: immunization characteristics and potentials of blockingIL-33/ST2 pathway in allergic airway inflammation.Hum Vaccin Immunother. 2014;10(8):2303-11. doi: 10.4161/hv.29425. Hum Vaccin Immunother. 2014. PMID: 25424936 Free PMC article.
References
-
- Boulet LP, et al. Airway hyperresponsiveness, inflammation and subepithelial collagen deposition in recently diagnosed versus long-standing mild asthma. Influence of inhaled corticosteroids. Am. J. Respir. Crit. Care Med. 2000;162:1308–1313. - PubMed
-
- Chakir J, et al. Airway remodeling-associated mediators in moderate to severe asthma: effect of steroids on TGF-β, IL-11, IL-17 and type I and type III collagen expression. J. Allergy Clin. Immunol. 2003;111:1293–1298. - PubMed
-
- Pepe C, et al. Differences in airway remodeling between subjects with severe and moderate asthma. J. Allergy Clin. Immunol. 2005;116:544–549. - PubMed
-
- Wills-Karp M. Interleukin-13 in asthma pathogenesis. Immunol. Rev. 2004;202:175–190. - PubMed
-
- Halwani R, Al-Muhsen S, Al-Jahdali H, Hamid Q. Role of transforming growth factor-β in airway remodeling in asthma. Am. J. Respir. Cell Mol. Biol. 2011;44:127–133. - PubMed
Publication types
MeSH terms
Substances
Grants and funding
- AI067890/AI/NIAID NIH HHS/United States
- 1K08AI080938-01A1/AI/NIAID NIH HHS/United States
- R37 AI038425/AI/NIAID NIH HHS/United States
- R01 AI067890-06/AI/NIAID NIH HHS/United States
- K08 AI080938/AI/NIAID NIH HHS/United States
- R01 AI048073/AI/NIAID NIH HHS/United States
- R37AI068033/AI/NIAID NIH HHS/United States
- U19 AI070535/AI/NIAID NIH HHS/United States
- R01 AI067890/AI/NIAID NIH HHS/United States
- R37 AI033068-21/AI/NIAID NIH HHS/United States
- R01 AI068033/AI/NIAID NIH HHS/United States
- U19 AI070535-05/AI/NIAID NIH HHS/United States
- R37 AI033068/AI/NIAID NIH HHS/United States
- K08 AI080938-01A1/AI/NIAID NIH HHS/United States
- AI070535/AI/NIAID NIH HHS/United States
LinkOut - more resources
Full Text Sources
Other Literature Sources
Medical
Molecular Biology Databases