The SCHOOL of nature: III. From mechanistic understanding to novel therapies
- PMID: 21487477
- PMCID: PMC3047783
- DOI: 10.4161/self.1.3.12794
The SCHOOL of nature: III. From mechanistic understanding to novel therapies
Abstract
Protein-protein interactions play a central role in biological processes and thus represent an appealing target for innovative drug design and development. They can be targeted by small molecule inhibitors, modulatory peptides and peptidomimetics, which represent a superior alternative to protein therapeutics that carry many disadvantages. Considering that transmembrane signal transduction is an attractive process to therapeutically control multiple diseases, it is fundamentally and clinically important to mechanistically understand how signal transduction occurs. Uncovering specific protein-protein interactions critical for signal transduction, a general platform for receptor-mediated signaling, the signaling chain homooligomerization (SCHOOL) platform, suggests these interactions as universal therapeutic targets. Within the platform, the general principles of signaling are similar for a variety of functionally unrelated receptors. This suggests that global therapeutic strategies targeting key protein-protein interactions involved in receptor triggering and transmembrane signal transduction may be used to treat a diverse set of diseases. This also assumes that clinical knowledge and therapeutic strategies can be transferred between seemingly disparate disorders, such as T cell-mediated skin diseases and platelet disorders or combined to develop novel pharmacological approaches. Intriguingly, human viruses use the SCHOOL-like strategies to modulate and/or escape the host immune response. These viral mechanisms are highly optimized over the millennia, and the lessons learned from viral pathogenesis can be used practically for rational drug design. Proof of the SCHOOL concept in the development of novel therapies for atopic dermatitis, rheumatoid arthritis, cancer, platelet disorders and other multiple indications with unmet needs opens new horizons in therapeutics.
Figures
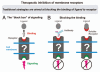
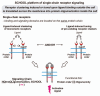
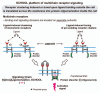
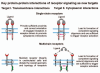
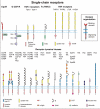
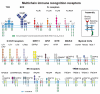
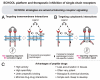
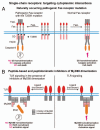
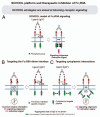
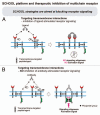
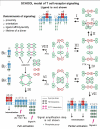
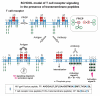
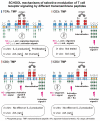
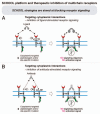
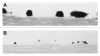
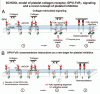
Similar articles
-
SCHOOL model and new targeting strategies.Adv Exp Med Biol. 2008;640:268-311. doi: 10.1007/978-0-387-09789-3_20. Adv Exp Med Biol. 2008. PMID: 19065798 Free PMC article. Review.
-
Targeting Intramembrane Protein-Protein Interactions: Novel Therapeutic Strategy of Millions Years Old.Adv Protein Chem Struct Biol. 2018;111:61-99. doi: 10.1016/bs.apcsb.2017.06.004. Epub 2017 Jul 24. Adv Protein Chem Struct Biol. 2018. PMID: 29459036 Free PMC article. Review.
-
Transmembrane interactions as immunotherapeutic targets: lessons from viral pathogenesis.Adv Exp Med Biol. 2007;601:335-44. doi: 10.1007/978-0-387-72005-0_36. Adv Exp Med Biol. 2007. PMID: 17713022 Review.
-
New therapeutic strategies targeting transmembrane signal transduction in the immune system.Cell Adh Migr. 2010 Apr-Jun;4(2):255-67. doi: 10.4161/cam.4.2.10746. Epub 2010 Apr 24. Cell Adh Migr. 2010. PMID: 20519929 Free PMC article. Review.
-
The SCHOOL of nature: I. Transmembrane signaling.Self Nonself. 2010 Jan;1(1):4-39. doi: 10.4161/self.1.1.10832. Self Nonself. 2010. PMID: 21559175 Free PMC article.
Cited by
-
SARS Coronavirus Fusion Peptide-Derived Sequence Suppresses Collagen-Induced Arthritis in DBA/1J Mice.Sci Rep. 2016 Jun 28;6:28672. doi: 10.1038/srep28672. Sci Rep. 2016. PMID: 27349522 Free PMC article.
-
Unusual biophysics of immune signaling-related intrinsically disordered proteins.Self Nonself. 2010 Oct;1(4):271-281. doi: 10.4161/self.1.4.13641. Self Nonself. 2010. PMID: 21487502 Free PMC article.
-
Inhibition of TREM-2 Markedly Suppresses Joint Inflammation and Damage in Experimental Arthritis.Int J Mol Sci. 2022 Aug 9;23(16):8857. doi: 10.3390/ijms23168857. Int J Mol Sci. 2022. PMID: 36012120 Free PMC article.
-
Cells diversify transmembrane signaling through the controlled chaos of protein disorder.Self Nonself. 2011 Apr;2(2):75-79. doi: 10.4161/self.2.2.15756. Epub 2011 Apr 1. Self Nonself. 2011. PMID: 22299058 Free PMC article.
-
The SCHOOL of nature: IV. Learning from viruses.Self Nonself. 2010 Oct;1(4):282-298. doi: 10.4161/self.1.4.13279. Self Nonself. 2010. PMID: 21487503 Free PMC article.
References
-
- Fry DC. Protein-protein interactions as targets for small molecule drug discovery. Biopolymers. 2006;84:535–552. - PubMed
-
- Ryan DP, Matthews JM. Protein-protein interactions in human disease. Curr Opin Struct Biol. 2005;15:441–446. - PubMed
-
- Toogood PL. Inhibition of protein-protein association by small molecules: approaches and progress. J Med Chem. 2002;45:1543–1558. - PubMed
-
- Hershberger SJ, Lee SG, Chmielewski J. Scaffolds for blocking protein-protein interactions. Curr Top Med Chem. 2007;7:928–942. - PubMed
LinkOut - more resources
Full Text Sources
Other Literature Sources