Partial pneumonectomy of telomerase null mice carrying shortened telomeres initiates cell growth arrest resulting in a limited compensatory growth response
- PMID: 21460122
- PMCID: PMC3119124
- DOI: 10.1152/ajplung.00409.2010
Partial pneumonectomy of telomerase null mice carrying shortened telomeres initiates cell growth arrest resulting in a limited compensatory growth response
Abstract
Telomerase mutations and significantly shortened chromosomal telomeres have recently been implicated in human lung pathologies. Natural telomere shortening is an inevitable consequence of aging, which is also a risk factor for development of lung disease. However, the impact of shortened telomeres and telomerase dysfunction on the ability of lung cells to respond to significant challenge is still largely unknown. We have previously shown that lungs of late generation, telomerase null B6.Cg-Terc(tm1Rdp) mice feature alveolar simplification and chronic stress signaling at baseline, a phenocopy of aged lung. To determine the role telomerase plays when the lung is challenged, B6.Cg-Terc(tm1Rdp) mice carrying shortened telomeres and wild-type controls were subjected to partial pneumonectomy. We found that telomerase activity was strongly induced in alveolar epithelial type 2 cells (AEC2) of the remaining lung immediately following surgery. Eighty-six percent of wild-type animals survived the procedure and exhibited a burst of early compensatory growth marked by upregulation of proliferation, stress response, and DNA repair pathways in AEC2. In B6.Cg-Terc(tm1Rdp) mice carrying shortened telomeres, response to pneumonectomy was characterized by decreased survival, diminished compensatory lung growth, attenuated distal lung progenitor cell response, persistent DNA damage, and cell growth arrest. Overall, survival correlated strongly with telomere length. We conclude that functional telomerase and properly maintained telomeres play key roles in both long-term survival and the early phase of compensatory lung growth following partial pneumonectomy.
Figures
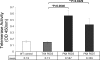
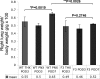
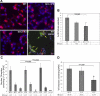
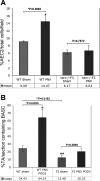
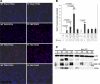
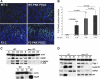
Similar articles
-
Pulmonary Alveolar Stem Cell Senescence, Apoptosis, and Differentiation by p53-Dependent and -Independent Mechanisms in Telomerase-Deficient Mice.Cells. 2021 Oct 26;10(11):2892. doi: 10.3390/cells10112892. Cells. 2021. PMID: 34831112 Free PMC article.
-
Telomerase Deficiency Causes Alveolar Stem Cell Senescence-associated Low-grade Inflammation in Lungs.J Biol Chem. 2015 Dec 25;290(52):30813-29. doi: 10.1074/jbc.M115.681619. Epub 2015 Oct 30. J Biol Chem. 2015. PMID: 26518879 Free PMC article.
-
Lung alveolar integrity is compromised by telomere shortening in telomerase-null mice.Am J Physiol Lung Cell Mol Physiol. 2009 Jan;296(1):L57-70. doi: 10.1152/ajplung.90411.2008. Epub 2008 Oct 24. Am J Physiol Lung Cell Mol Physiol. 2009. PMID: 18952756 Free PMC article.
-
Control of Cellular Aging, Tissue Function, and Cancer by p53 Downstream of Telomeres.Cold Spring Harb Perspect Med. 2017 May 1;7(5):a026088. doi: 10.1101/cshperspect.a026088. Cold Spring Harb Perspect Med. 2017. PMID: 28289249 Free PMC article. Review.
-
Telomeres, telomerase and chromosome stability.Radiat Res. 1997 May;147(5):529-34. Radiat Res. 1997. PMID: 9146697 Review.
Cited by
-
Age dependence of lung mesenchymal stromal cell dynamics following pneumonectomy.Stem Cells Dev. 2013 Dec 15;22(24):3214-25. doi: 10.1089/scd.2012.0477. Epub 2013 Aug 30. Stem Cells Dev. 2013. PMID: 23895415 Free PMC article.
-
Pulmonary Alveolar Stem Cell Senescence, Apoptosis, and Differentiation by p53-Dependent and -Independent Mechanisms in Telomerase-Deficient Mice.Cells. 2021 Oct 26;10(11):2892. doi: 10.3390/cells10112892. Cells. 2021. PMID: 34831112 Free PMC article.
-
Senescence in chronic obstructive pulmonary disease.Proc Am Thorac Soc. 2012 May;9(2):62-3. doi: 10.1513/pats.201201-012MS. Proc Am Thorac Soc. 2012. PMID: 22550244 Free PMC article. Review.
-
Telomerase Deficiency Causes Alveolar Stem Cell Senescence-associated Low-grade Inflammation in Lungs.J Biol Chem. 2015 Dec 25;290(52):30813-29. doi: 10.1074/jbc.M115.681619. Epub 2015 Oct 30. J Biol Chem. 2015. PMID: 26518879 Free PMC article.
-
Telomerase deficiency does not alter bleomycin-induced fibrosis in mice.Exp Lung Res. 2012 Apr;38(3):124-34. doi: 10.3109/01902148.2012.658148. Exp Lung Res. 2012. PMID: 22394286 Free PMC article.
References
-
- Adamson YR, Bowden DH. The Type 2 cell as progenitor of alveolar epithelial regeneration. A cytodynamic study in mice after exposure to oxygen. Lab Invest 30: 35–42, 1974 - PubMed
-
- Armanios M, Chen JL, Chang YPC, Brodsky RA, Hawkins A, Griffin CA, Eshleman JR, Cohen AR, Chakravarti A, Hamosh A, Greider CW. Haploinsufficiency of telomerase reverse transcriptase leads to anticipation in autosomal dominant dyskeratosis congenita. Proc Natl Acad Sci USA 102: 15960–15964, 2005 - PMC - PubMed
-
- Armanios MY, Chen JJ, Cogan JD, Alder JK, Ingersoll RG, Markin C, Lawson WE, Xie M, Vulto I, Phillips JA, 3rd, Lansdorp PM, Greider CW, Loyd JE. Telomerase mutations in families with idiopathic pulmonary fibrosis. N Engl J Med 356: 1317–1326, 2007 - PubMed
Publication types
MeSH terms
Substances
Grants and funding
LinkOut - more resources
Full Text Sources
Medical
Molecular Biology Databases