Arrestin-rhodopsin binding stoichiometry in isolated rod outer segment membranes depends on the percentage of activated receptors
- PMID: 21169358
- PMCID: PMC3044992
- DOI: 10.1074/jbc.M110.204941
Arrestin-rhodopsin binding stoichiometry in isolated rod outer segment membranes depends on the percentage of activated receptors
Abstract
In the rod cell of the retina, arrestin is responsible for blocking signaling of the G-protein-coupled receptor rhodopsin. The general visual signal transduction model implies that arrestin must be able to interact with a single light-activated, phosphorylated rhodopsin molecule (Rho*P), as would be generated at physiologically relevant low light levels. However, the elongated bi-lobed structure of arrestin suggests that it might be able to accommodate two rhodopsin molecules. In this study, we directly addressed the question of binding stoichiometry by quantifying arrestin binding to Rho*P in isolated rod outer segment membranes. We manipulated the "photoactivation density," i.e. the percentage of active receptors in the membrane, with the use of a light flash or by partially regenerating membranes containing phosphorylated opsin with 11-cis-retinal. Curiously, we found that the apparent arrestin-Rho*P binding stoichiometry was linearly dependent on the photoactivation density, with one-to-one binding at low photoactivation density and one-to-two binding at high photoactivation density. We also observed that, irrespective of the photoactivation density, a single arrestin molecule was able to stabilize the active metarhodopsin II conformation of only a single Rho*P. We hypothesize that, although arrestin requires at least a single Rho*P to bind the membrane, a single arrestin can actually interact with a pair of receptors. The ability of arrestin to interact with heterogeneous receptor pairs composed of two different photo-intermediate states would be well suited to the rod cell, which functions at low light intensity but is routinely exposed to several orders of magnitude more light.
Figures
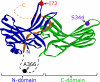
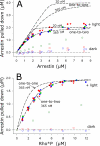
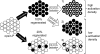
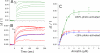
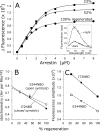
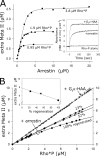
Similar articles
-
Formation and decay of the arrestin·rhodopsin complex in native disc membranes.J Biol Chem. 2015 May 15;290(20):12919-28. doi: 10.1074/jbc.M114.620898. Epub 2015 Apr 6. J Biol Chem. 2015. PMID: 25847250 Free PMC article.
-
Constitutively active rhodopsin mutants causing night blindness are effectively phosphorylated by GRKs but differ in arrestin-1 binding.Cell Signal. 2013 Nov;25(11):2155-62. doi: 10.1016/j.cellsig.2013.07.009. Epub 2013 Jul 17. Cell Signal. 2013. PMID: 23872075 Free PMC article.
-
Involvement of distinct arrestin-1 elements in binding to different functional forms of rhodopsin.Proc Natl Acad Sci U S A. 2013 Jan 15;110(3):942-7. doi: 10.1073/pnas.1215176110. Epub 2012 Dec 31. Proc Natl Acad Sci U S A. 2013. PMID: 23277586 Free PMC article.
-
Rhodopsin phosphorylation: from terminating single photon responses to photoreceptor dark adaptation.Trends Neurosci. 2002 Mar;25(3):124-6. doi: 10.1016/s0166-2236(00)02094-4. Trends Neurosci. 2002. PMID: 11852136 Review.
-
Control of rhodopsin activity in vision.Eye (Lond). 1998;12 ( Pt 3b):521-5. doi: 10.1038/eye.1998.140. Eye (Lond). 1998. PMID: 9775212 Review.
Cited by
-
Critical role of the central 139-loop in stability and binding selectivity of arrestin-1.J Biol Chem. 2013 Apr 26;288(17):11741-50. doi: 10.1074/jbc.M113.450031. Epub 2013 Mar 8. J Biol Chem. 2013. PMID: 23476014 Free PMC article.
-
Crystal structure of a common GPCR-binding interface for G protein and arrestin.Nat Commun. 2014 Sep 10;5:4801. doi: 10.1038/ncomms5801. Nat Commun. 2014. PMID: 25205354 Free PMC article.
-
The functional cycle of visual arrestins in photoreceptor cells.Prog Retin Eye Res. 2011 Nov;30(6):405-30. doi: 10.1016/j.preteyeres.2011.07.002. Epub 2011 Jul 29. Prog Retin Eye Res. 2011. PMID: 21824527 Free PMC article. Review.
-
Formation and decay of the arrestin·rhodopsin complex in native disc membranes.J Biol Chem. 2015 May 15;290(20):12919-28. doi: 10.1074/jbc.M114.620898. Epub 2015 Apr 6. J Biol Chem. 2015. PMID: 25847250 Free PMC article.
-
Constitutively active rhodopsin mutants causing night blindness are effectively phosphorylated by GRKs but differ in arrestin-1 binding.Cell Signal. 2013 Nov;25(11):2155-62. doi: 10.1016/j.cellsig.2013.07.009. Epub 2013 Jul 17. Cell Signal. 2013. PMID: 23872075 Free PMC article.
References
-
- Hofmann K. P., Scheerer P., Hildebrand P. W., Choe H. W., Park J. H., Heck M., Ernst O. P. (2009) Trends Biochem. Sci. 34, 540–552 - PubMed
-
- Palczewski K., Kumasaka T., Hori T., Behnke C. A., Motoshima H., Fox B. A., Le Trong I., Teller D. C., Okada T., Stenkamp R. E., Yamamoto M., Miyano M. (2000) Science 289, 739–745 - PubMed
Publication types
MeSH terms
Substances
LinkOut - more resources
Full Text Sources