Tunneling-nanotube development in astrocytes depends on p53 activation
- PMID: 21113142
- PMCID: PMC3131904
- DOI: 10.1038/cdd.2010.147
Tunneling-nanotube development in astrocytes depends on p53 activation
Abstract
Tunneling nanotubes (TNTs) can be induced in rat hippocampal astrocytes and neurons with H(2)O(2) or serum depletion. Major cytoskeletal component of TNTs is F-actin. TNTs transfer endoplasmic reticulum, mitochondria, Golgi, endosome and intracellular as well as extracellular amyloid β. TNT development is a property of cells under stress. When two populations of cells are co-cultured, it is the stressed cells that always develop TNTs toward the unstressed cells. p53 is crucial for TNT development. When p53 function is deleted by either dominant negative construct or siRNAs, TNT development is inhibited. In addition, we find that among the genes activated by p53, epidermal growth factor receptor is also important to TNT development. Akt, phosphoinositide 3-kinase and mTOR are involved in TNT induction. Our data suggest that TNTs might be a mechanism for cells to respond to harmful signals and transfer cellular substances or energy to another cell under stress.
© 2011 Macmillan Publishers Limited
Figures
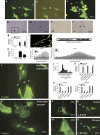
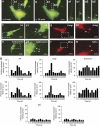
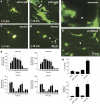
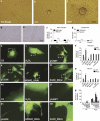
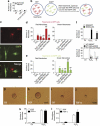
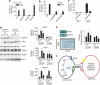
Similar articles
-
Stress-induced tunneling nanotubes support treatment adaptation in prostate cancer.Sci Rep. 2019 May 24;9(1):7826. doi: 10.1038/s41598-019-44346-5. Sci Rep. 2019. PMID: 31127190 Free PMC article.
-
ROS/mtROS promotes TNTs formation via the PI3K/AKT/mTOR pathway to protect against mitochondrial damages in glial cells induced by engineered nanomaterials.Part Fibre Toxicol. 2024 Jan 15;21(1):1. doi: 10.1186/s12989-024-00562-0. Part Fibre Toxicol. 2024. PMID: 38225661 Free PMC article.
-
Tunneling nanotubes between rat primary astrocytes and C6 glioma cells alter proliferation potential of glioma cells.Neurosci Bull. 2015 Jun;31(3):371-8. doi: 10.1007/s12264-014-1522-4. Epub 2015 Apr 26. Neurosci Bull. 2015. PMID: 25913038 Free PMC article.
-
Tunneling nanotubes: The transport highway for astrocyte-neuron communication in the central nervous system.Brain Res Bull. 2024 Apr;209:110921. doi: 10.1016/j.brainresbull.2024.110921. Epub 2024 Mar 5. Brain Res Bull. 2024. PMID: 38447659 Review.
-
Wiring through tunneling nanotubes--from electrical signals to organelle transfer.J Cell Sci. 2012 Mar 1;125(Pt 5):1089-98. doi: 10.1242/jcs.083279. Epub 2012 Mar 7. J Cell Sci. 2012. PMID: 22399801 Review.
Cited by
-
Developing neurons form transient nanotubes facilitating electrical coupling and calcium signaling with distant astrocytes.PLoS One. 2012;7(10):e47429. doi: 10.1371/journal.pone.0047429. Epub 2012 Oct 11. PLoS One. 2012. PMID: 23071805 Free PMC article.
-
Identification of NCF2/p67phox as a novel p53 target gene.Cell Cycle. 2012 Dec 15;11(24):4589-96. doi: 10.4161/cc.22853. Epub 2012 Nov 27. Cell Cycle. 2012. PMID: 23187810 Free PMC article.
-
Can Wharton jelly derived or adipose tissue derived mesenchymal stem cell can be a treatment option for duchenne muscular dystrophy? Answers as transcriptomic aspect.Am J Stem Cells. 2020 Aug 25;9(4):57-67. eCollection 2020. Am J Stem Cells. 2020. PMID: 32929392 Free PMC article.
-
Prion aggregates transfer through tunneling nanotubes in endocytic vesicles.Prion. 2015;9(2):125-35. doi: 10.1080/19336896.2015.1025189. Prion. 2015. PMID: 25996400 Free PMC article.
-
Direct Cell-Cell Communication via Membrane Pores, Gap Junction Channels, and Tunneling Nanotubes: Medical Relevance of Mitochondrial Exchange.Int J Mol Sci. 2022 May 30;23(11):6133. doi: 10.3390/ijms23116133. Int J Mol Sci. 2022. PMID: 35682809 Free PMC article. Review.
References
-
- Rustom A, Saffrich R, Markovic I, Walther P, Gerdes HH. Nanotubular highways for intercellular organelle transport. Science. 2004;303:1007–1010. - PubMed
-
- Onfelt B, Davis DM. Can membrane nanotubes facilitate communication between immune cells. Biochem Soc Trans. 2004;32 (Part 5:676–678. - PubMed
-
- Onfelt B, Nedvetzki S, Yanagi K, Davis DM. Cutting edge: membrane nanotubes connect immune cells. J Immunol. 2004;173:1511–1513. - PubMed
-
- Watkins SC, Salter RD. Functional connectivity between immune cells mediated by tunneling nanotubules. Immunity. 2005;23:309–318. - PubMed
Publication types
MeSH terms
Substances
LinkOut - more resources
Full Text Sources
Other Literature Sources
Research Materials
Miscellaneous