Recombination of 5' subgenomic RNA3a with genomic RNA3 of Brome mosaic bromovirus in vitro and in vivo
- PMID: 21111438
- PMCID: PMC7111948
- DOI: 10.1016/j.virol.2010.10.037
Recombination of 5' subgenomic RNA3a with genomic RNA3 of Brome mosaic bromovirus in vitro and in vivo
Abstract
RNA-RNA recombination salvages viral RNAs and contributes to their genomic variability. A recombinationally-active subgenomic promoter (sgp) has been mapped in Brome mosaic bromovirus (BMV) RNA3 (Wierzchoslawski et al., 2004. J. Virol.78, 8552-8864) and mRNA-like 5' sgRNA3a was characterized (Wierzchoslawski et al., 2006. J. Virol. 80, 12357-12366). In this paper we describe sgRNA3a-mediated recombination in both in vitro and in vivo experiments. BMV replicase-directed co-copying of (-) RNA3 with wt sgRNA3a generated RNA3 recombinants in vitro, but it failed to when 3'-truncated sgRNA3a was substituted, demonstrating a role for the 3' polyA tail. Barley protoplast co-transfections revealed that (i) wt sgRNA3a recombines at the 3' and the internal sites; (ii) 3'-truncated sgRNA3as recombine more upstream; and (iii) 5'-truncated sgRNA3 recombine at a low rate. In planta co-inoculations confirmed the RNA3-sgRNA3a crossovers. In summary, the non-replicating sgRNA3a recombines with replicating RNA3, most likely via primer extension and/or internal template switching.
Copyright © 2010 Elsevier Inc. All rights reserved.
Figures
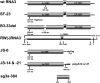
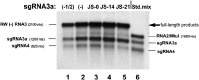
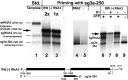
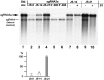
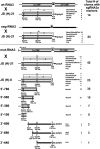
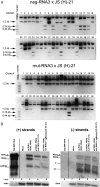

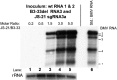
Similar articles
-
Characterization of a novel 5' subgenomic RNA3a derived from RNA3 of Brome mosaic bromovirus.J Virol. 2006 Dec;80(24):12357-66. doi: 10.1128/JVI.01207-06. Epub 2006 Sep 27. J Virol. 2006. PMID: 17005659 Free PMC article.
-
A transcriptionally active subgenomic promoter supports homologous crossovers in a plus-strand RNA virus.J Virol. 2003 Jun;77(12):6769-76. doi: 10.1128/jvi.77.12.6769-6776.2003. J Virol. 2003. PMID: 12767997 Free PMC article.
-
Efficient in vitro system of homologous recombination in brome mosaic bromovirus.J Virol. 2006 Jun;80(12):6182-7. doi: 10.1128/JVI.02447-05. J Virol. 2006. PMID: 16731958 Free PMC article.
-
RNA recombination in brome mosaic virus, a model plus strand RNA virus.Acta Biochim Pol. 1998;45(4):847-68. Acta Biochim Pol. 1998. PMID: 10397334 Review.
-
The coat protein leads the way: an update on basic and applied studies with the Brome mosaic virus coat protein.Mol Plant Pathol. 2011 May;12(4):403-12. doi: 10.1111/j.1364-3703.2010.00678.x. Epub 2010 Nov 25. Mol Plant Pathol. 2011. PMID: 21453435 Free PMC article. Review.
Cited by
-
Intermolecular RNA Recombination Occurs at Different Frequencies in Alternate Forms of Brome Mosaic Virus RNA Replication Compartments.Viruses. 2018 Mar 15;10(3):131. doi: 10.3390/v10030131. Viruses. 2018. PMID: 29543718 Free PMC article.
-
Co-infection with two strains of Brome mosaic bromovirus reveals common RNA recombination sites in different hosts.Virus Evol. 2015 Dec 23;1(1):vev021. doi: 10.1093/ve/vev021. eCollection 2015. Virus Evol. 2015. PMID: 27774290 Free PMC article.
-
Emergence of distinct brome mosaic virus recombinants is determined by the polarity of the inoculum RNA.J Virol. 2012 May;86(9):5204-20. doi: 10.1128/JVI.00351-12. Epub 2012 Feb 22. J Virol. 2012. PMID: 22357282 Free PMC article.
-
Mutations in the coat protein-binding cis-acting RNA motifs debilitate RNA recombination of Brome mosaic virus.Virus Res. 2012 Dec;170(1-2):138-49. doi: 10.1016/j.virusres.2012.10.001. Epub 2012 Oct 16. Virus Res. 2012. PMID: 23079110 Free PMC article.
-
Subgenomic messenger RNAs: mastering regulation of (+)-strand RNA virus life cycle.Virology. 2011 Apr 10;412(2):245-55. doi: 10.1016/j.virol.2011.02.007. Epub 2011 Mar 5. Virology. 2011. PMID: 21377709 Free PMC article. Review.
References
-
- Adair T.L., Kearney C.M. Recombination between a 3-kilobase Tobacco mosaic virus transgene and a homologous viral construct in the restoration of viral and nonviral genes. Arch. Virol. 2000;145:1867–1883. - PubMed
-
- Agol V.I. Molecular mechanisms of Poliovirus variation and evolution. Curr. Top. Microbiol. Immunol. 2006;299:211–259. - PubMed
-
- Alejska M., Kurzyńska-Kokorniak A., Broda M., Kierzek R., Figlerowicz M. How RNA viruses exchange their genetic material. Acta Biochim. Pol. 2001;48:391–407. - PubMed
-
- Allison R., Janda M., Ahlquist P. Sequence of Cowpea chlorotic mottle virus RNAs 2 and 3 and evidence of a recombination event during bromoviral evolution. Virology. 1989;172:321–330. - PubMed
Publication types
MeSH terms
Substances
Grants and funding
LinkOut - more resources
Full Text Sources