Emerging roles for XBP1, a sUPeR transcription factor
- PMID: 21061914
- PMCID: PMC3374844
- DOI: 10.3727/105221610x12819686555051
Emerging roles for XBP1, a sUPeR transcription factor
Abstract
X-box binding protein 1 (XBP1) is a unique basic region leucine zipper (bZIP) transcription factor whose active form is generated by a nonconventional splicing reaction upon disruption of homeostasis in the endoplasmic reticulum (ER) and activation of the unfolded protein response (UPR). XBP1, first identified as a key regulator of major histocompatibility complex (MHC) class II gene expression in B cells, represents the most conserved signaling component of UPR and is critical for cell fate determination in response to ER stress. Here we review recent advances in our understanding of this multifaceted transcription factor in health and diseases.
Figures
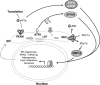
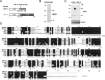
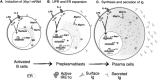
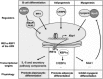
Similar articles
-
The emerging role of XBP1 in cancer.Biomed Pharmacother. 2020 Jul;127:110069. doi: 10.1016/j.biopha.2020.110069. Epub 2020 Apr 12. Biomed Pharmacother. 2020. PMID: 32294597 Review.
-
A conformational RNA zipper promotes intron ejection during non-conventional XBP1 mRNA splicing.EMBO Rep. 2015 Dec;16(12):1688-98. doi: 10.15252/embr.201540955. Epub 2015 Oct 19. EMBO Rep. 2015. PMID: 26483401 Free PMC article.
-
The unfolded protein response (UPR)-activated transcription factor X-box-binding protein 1 (XBP1) induces microRNA-346 expression that targets the human antigen peptide transporter 1 (TAP1) mRNA and governs immune regulatory genes.J Biol Chem. 2011 Dec 2;286(48):41862-41870. doi: 10.1074/jbc.M111.304956. Epub 2011 Oct 14. J Biol Chem. 2011. PMID: 22002058 Free PMC article.
-
The balance between adaptive and apoptotic unfolded protein responses regulates β-cell death under ER stress conditions through XBP1, CHOP and JNK.Mol Cell Endocrinol. 2015 Sep 15;413:189-201. doi: 10.1016/j.mce.2015.06.025. Epub 2015 Jun 30. Mol Cell Endocrinol. 2015. PMID: 26135354
-
Targeting the IRE1α-XBP1 branch of the unfolded protein response in human diseases.Semin Cancer Biol. 2015 Aug;33:48-56. doi: 10.1016/j.semcancer.2015.04.010. Epub 2015 May 16. Semin Cancer Biol. 2015. PMID: 25986851 Free PMC article. Review.
Cited by
-
Single-Cell Sequencing of Immune Cells in Human Aortic Dissection Tissue Provides Insights Into Immune Cell Heterogeneity.Front Cardiovasc Med. 2022 Mar 31;9:791875. doi: 10.3389/fcvm.2022.791875. eCollection 2022. Front Cardiovasc Med. 2022. PMID: 35433892 Free PMC article.
-
Dual RNase activity of IRE1 as a target for anticancer therapies.J Cell Commun Signal. 2023 Dec;17(4):1145-1161. doi: 10.1007/s12079-023-00784-5. Epub 2023 Sep 18. J Cell Commun Signal. 2023. PMID: 37721642 Free PMC article. Review.
-
A small molecule UPR modulator for diabetes identified by high throughput screening.Acta Pharm Sin B. 2021 Dec;11(12):3983-3993. doi: 10.1016/j.apsb.2021.05.018. Epub 2021 Jun 16. Acta Pharm Sin B. 2021. PMID: 35024320 Free PMC article.
-
The transcription factor XBP1 in memory and cognition: Implications in Alzheimer disease.Mol Med. 2017 Feb;22:905-917. doi: 10.2119/molmed.2016.00229. Epub 2017 Jan 4. Mol Med. 2017. PMID: 28079229 Free PMC article.
-
A Bovine Lymphosarcoma Cell Line Infected with Theileria annulata Exhibits an Irreversible Reconfiguration of Host Cell Gene Expression.PLoS One. 2013 Jun 26;8(6):e66833. doi: 10.1371/journal.pone.0066833. Print 2013. PLoS One. 2013. PMID: 23840536 Free PMC article.
References
-
- Acosta-Alvear D.; Zhou Y.; Blais A.; Tsikitis M.; Lents N. H.; Arias C.; Lennon C. J.; Kluger Y.; Dynlacht B. D. XBP1 controls diverse cell type- and condition-specific transcriptional regulatory networks. Mol. Cell 27:53–66; 2007. - PubMed
-
- Barmada M. M.; Brant S. R.; Nicolae D. L.; Achkar J. P.; Panhuysen C. I.; Bayless T. M.; Cho J. H.; Duerr R. H. A genome scan in 260 inflammatory bowel disease-affected relative pairs. Inflamm. Bowel Dis. 10:513–520; 2004. - PubMed
-
- Brant S. R.; Fu Y.; Fields C. T.; Baltazar R.; Ravenhill G.; Pickles M. R.; Rohal P. M.; Mann J.; Kirschner B. S.; Jabs E. W.; Bayless T. M.; Hanauer S. B.; Cho J. H. American families with Crohn’s disease have strong evidence for linkage to chromosome 16 but not chromosome 12. Gastroenterology 115:1056–1061; 1998. - PubMed
Publication types
MeSH terms
Substances
Grants and funding
LinkOut - more resources
Full Text Sources
Other Literature Sources
Research Materials