Use of base modifications in primers and amplicons to improve nucleic acids detection in the real-time snake polymerase chain reaction
- PMID: 21050073
- PMCID: PMC3033208
- DOI: 10.1089/adt.2010.0303
Use of base modifications in primers and amplicons to improve nucleic acids detection in the real-time snake polymerase chain reaction
Abstract
The addition of relatively short flap sequence at the 5'-end of one of the polymerase chain reaction (PCR) primers considerably improves performance of real-time assays based on 5'-nuclease activity. This new technology, called Snake, was shown to supersede the conventional methods like TaqMan, Molecular Beacons, and Scorpions in the signal productivity and discrimination of target polymorphic variations as small as single nucleotides. The present article describes a number of reaction conditions and methods that allow further improvement of the assay performance. One of the identified approaches is the use of duplex-destabilizing modifications such as deoxyinosine and deoxyuridine in the design of the Snake primers. This approach was shown to solve the most serious problem associated with the antisense amplicon folding and cleavage. As a result, the method permits the use of relatively long-in this study-14-mer flap sequences. Investigation also revealed that only the 5'-segment of the flap requires the deoxyinosine/deoxyuridine destabilization, whereas the 3'-segment is preferably left unmodified or even stabilized using 2-amino deoxyadenosine d(2-amA) and 5-propynyl deoxyuridine d(5-PrU) modifications. The base-modification technique is especially effective when applied in combination with asymmetric three-step PCR. The most valuable discovery of the present study is the effective application of modified deoxynucleoside 5'-triphosphates d(2-amA)TP and d(5-PrU)TP in Snake PCR. This method made possible the use of very short 6-8-mer 5'-flap sequences in Snake primers.
Figures
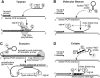
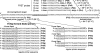
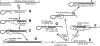
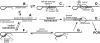
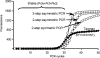
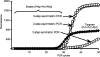
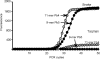
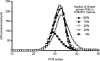
Similar articles
-
New approach to real-time nucleic acids detection: folding polymerase chain reaction amplicons into a secondary structure to improve cleavage of Forster resonance energy transfer probes in 5'-nuclease assays.Nucleic Acids Res. 2010 Mar;38(5):e29. doi: 10.1093/nar/gkp1138. Epub 2009 Dec 7. Nucleic Acids Res. 2010. PMID: 19969535 Free PMC article.
-
Use of base-modified duplex-stabilizing deoxynucleoside 5'-triphosphates to enhance the hybridization properties of primers and probes in detection polymerase chain reaction.Biochemistry. 2008 Dec 23;47(51):13666-73. doi: 10.1021/bi8017784. Biochemistry. 2008. PMID: 19046073
-
Electrochemical genosensor based on peptide nucleic acid-mediated PCR and asymmetric PCR techniques: Electrostatic interactions with a metal cation.Anal Chem. 2006 Apr 1;78(7):2182-9. doi: 10.1021/ac051526a. Anal Chem. 2006. PMID: 16579596
-
Homogeneous detection of nucleic acids using self-quenched polymerase chain reaction primers labeled with a single fluorophore (LUX primers).Methods Mol Biol. 2006;335:95-114. doi: 10.1385/1-59745-069-3:95. Methods Mol Biol. 2006. PMID: 16785623 Review.
-
High fidelity SNP genotyping using sequence-specific primer elongation and fluorescence correlation spectroscopy.Curr Pharm Biotechnol. 2003 Dec;4(6):477-84. doi: 10.2174/1389201033377391. Curr Pharm Biotechnol. 2003. PMID: 14683439 Review.
Cited by
-
Use of extremely short Förster resonance energy transfer probes in real-time polymerase chain reaction.Nucleic Acids Res. 2013 Nov;41(20):e191. doi: 10.1093/nar/gkt782. Epub 2013 Sep 5. Nucleic Acids Res. 2013. PMID: 24013564 Free PMC article.
References
-
- Higuchi R. Dollinger G. Walsh PS. Griffith R. Simultaneous amplification and detection of specific DNA sequences. Biotechnology. 1992;10:413–417. - PubMed
-
- Higuchi R. Fockler C. Dollinger G. Watson R. Kinetic PCR: real time monitoring of DNA amplification reactions. Biotechnology. 1993;11:1026–1030. - PubMed
-
- Förster T. Delocalized excitation, excitation transfer. In: Sinanoglu O, editor. Modern Quantum Chemistry, Istanbul Lectures, Part III. Academic Press; New York: 1965. pp. 93–137.
-
- Clegg RM. Fluorescence resonance energy transfer and nucleic acids. Methods Enzymol. 1992;211:353–388. - PubMed
Publication types
MeSH terms
Substances
Grants and funding
LinkOut - more resources
Full Text Sources
Other Literature Sources
Research Materials
Miscellaneous