Polypeptide in the chaperonin cage partly protrudes out and then folds inside or escapes outside
- PMID: 20959808
- PMCID: PMC3020636
- DOI: 10.1038/emboj.2010.262
Polypeptide in the chaperonin cage partly protrudes out and then folds inside or escapes outside
Abstract
The current mechanistic model of chaperonin-assisted protein folding assumes that the substrate protein in the cage, formed by GroEL central cavity capped with GroES, is isolated from outside and exists as a free polypeptide. However, using ATPase-deficient GroEL mutants that keep GroES bound, we found that, in the rate-limiting intermediate of a chaperonin reaction, the unfolded polypeptide in the cage partly protrudes through a narrow space near the GroEL/GroES interface. Then, the entire polypeptide is released either into the cage or to the outside medium. The former adopts a native structure very rapidly and the latter undergoes spontaneous folding. Partition of the in-cage folding and the escape varies among substrate proteins and is affected by hydrophobic interaction between the polypeptide and GroEL cavity wall. The ATPase-active GroEL with decreased in-cage folding produced less of a native model substrate protein in Escherichia coli cells. Thus, the polypeptide in the critical GroEL-GroES complex is neither free nor completely confined in the cage, but it is interacting with GroEL's apical region, partly protruding to outside.
Conflict of interest statement
The authors declare that they have no conflict of interest.
Figures
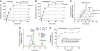
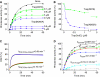
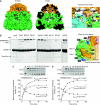
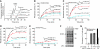
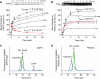
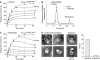
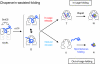
Similar articles
-
Productive folding of a tethered protein in the chaperonin GroEL-GroES cage.Biochem Biophys Res Commun. 2015 Oct 9;466(1):72-5. doi: 10.1016/j.bbrc.2015.08.108. Epub 2015 Aug 29. Biochem Biophys Res Commun. 2015. PMID: 26325470
-
GroEL-substrate-GroES ternary complexes are an important transient intermediate of the chaperonin cycle.J Biol Chem. 2002 Dec 27;277(52):50621-8. doi: 10.1074/jbc.M209183200. Epub 2002 Oct 10. J Biol Chem. 2002. PMID: 12377767
-
Mechanism of chaperonin action: GroES binding and release can drive GroEL-mediated protein folding in the absence of ATP hydrolysis.EMBO J. 1996 Nov 15;15(22):6111-21. EMBO J. 1996. PMID: 8947033 Free PMC article.
-
The GroEL-GroES Chaperonin Machine: A Nano-Cage for Protein Folding.Trends Biochem Sci. 2016 Jan;41(1):62-76. doi: 10.1016/j.tibs.2015.07.009. Epub 2015 Sep 25. Trends Biochem Sci. 2016. PMID: 26422689 Review.
-
Reaction Cycle of Chaperonin GroEL via Symmetric "Football" Intermediate.J Mol Biol. 2015 Sep 11;427(18):2912-8. doi: 10.1016/j.jmb.2015.04.007. Epub 2015 Apr 18. J Mol Biol. 2015. PMID: 25900372 Review.
Cited by
-
Chaperonin facilitates protein folding by avoiding initial polypeptide collapse.J Biochem. 2018 Nov 1;164(5):369-379. doi: 10.1093/jb/mvy061. J Biochem. 2018. PMID: 30053017 Free PMC article.
-
Probing water density and dynamics in the chaperonin GroEL cavity.J Am Chem Soc. 2014 Jul 2;136(26):9396-403. doi: 10.1021/ja503501x. Epub 2014 Jun 20. J Am Chem Soc. 2014. PMID: 24888581 Free PMC article.
-
Effects of C-terminal Truncation of Chaperonin GroEL on the Yield of In-cage Folding of the Green Fluorescent Protein.J Biol Chem. 2015 Jun 12;290(24):15042-51. doi: 10.1074/jbc.M114.633636. Epub 2015 Apr 17. J Biol Chem. 2015. PMID: 25887400 Free PMC article.
-
Temperature Regulates Stability, Ligand Binding (Mg2+ and ATP), and Stoichiometry of GroEL-GroES Complexes.J Am Chem Soc. 2022 Feb 16;144(6):2667-2678. doi: 10.1021/jacs.1c11341. Epub 2022 Feb 2. J Am Chem Soc. 2022. PMID: 35107280 Free PMC article.
-
Protein chain collapse modulation and folding stimulation by GroEL-ES.Sci Adv. 2022 Mar 4;8(9):eabl6293. doi: 10.1126/sciadv.abl6293. Epub 2022 Mar 4. Sci Adv. 2022. PMID: 35245117 Free PMC article.
References
-
- Ahrweiler PM, Frieden C (1991) Effects of point mutations in a hinge region on the stability, folding, and enzymatic activity of Escherichia coli dihydrofolate reductase. Biochemistry 30: 7801–7809 - PubMed
-
- Brinker A, Pfeifer G, Kerner MJ, Naylor DJ, Hartl FU, Hayer-Hartl M (2001) Dual function of protein confinement in chaperonin-assisted protein folding. Cell 107: 223–233 - PubMed
-
- Cliff MJ, Limpkin C, Cameron A, Burston SG, Clarke AR (2006) Elucidation of steps in the capture of a protein substrate for efficient encapsulation by GroE. J Biol Chem 281: 21266–21275 - PubMed
-
- Farr GW, Furtak K, Rowland MB, Ranson NA, Saibil HR, Kirchhausen T, Horwich AL (2000) Multivalent binding of nonnative substrate proteins by the chaperonin GroEL. Cell 100: 561–573 - PubMed
Publication types
MeSH terms
Substances
LinkOut - more resources
Full Text Sources
Molecular Biology Databases
Research Materials