Loss of maternal ATRX results in centromere instability and aneuploidy in the mammalian oocyte and pre-implantation embryo
- PMID: 20885787
- PMCID: PMC2944790
- DOI: 10.1371/journal.pgen.1001137
Loss of maternal ATRX results in centromere instability and aneuploidy in the mammalian oocyte and pre-implantation embryo
Abstract
The α-thalassemia/mental retardation X-linked protein (ATRX) is a chromatin-remodeling factor known to regulate DNA methylation at repetitive sequences of the human genome. We have previously demonstrated that ATRX binds to pericentric heterochromatin domains in mouse oocytes at the metaphase II stage where it is involved in mediating chromosome alignment at the meiotic spindle. However, the role of ATRX in the functional differentiation of chromatin structure during meiosis is not known. To test ATRX function in the germ line, we developed an oocyte-specific transgenic RNAi knockdown mouse model. Our results demonstrate that ATRX is required for heterochromatin formation and maintenance of chromosome stability during meiosis. During prophase I arrest, ATRX is necessary to recruit the transcriptional regulator DAXX (death domain associated protein) to pericentric heterochromatin. At the metaphase II stage, transgenic ATRX-RNAi oocytes exhibit abnormal chromosome morphology associated with reduced phosphorylation of histone 3 at serine 10 as well as chromosome segregation defects leading to aneuploidy and severely reduced fertility. Notably, a large proportion of ATRX-depleted oocytes and 1-cell stage embryos exhibit chromosome fragments and centromeric DNA-containing micronuclei. Our results provide novel evidence indicating that ATRX is required for centromere stability and the epigenetic control of heterochromatin function during meiosis and the transition to the first mitosis.
Conflict of interest statement
The authors have declared that no competing interests exist.
Figures
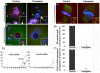
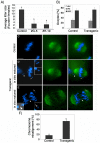
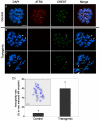
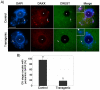
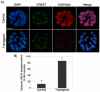
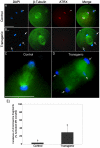
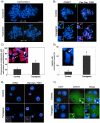
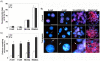
Similar articles
-
Chromatin structure and ATRX function in mouse oocytes.Results Probl Cell Differ. 2012;55:45-68. doi: 10.1007/978-3-642-30406-4_3. Results Probl Cell Differ. 2012. PMID: 22918800 Review.
-
ATRX, a member of the SNF2 family of helicase/ATPases, is required for chromosome alignment and meiotic spindle organization in metaphase II stage mouse oocytes.Dev Biol. 2004 Aug 1;272(1):1-14. doi: 10.1016/j.ydbio.2003.12.012. Dev Biol. 2004. PMID: 15242786
-
ATRX contributes to epigenetic asymmetry and silencing of major satellite transcripts in the maternal genome of the mouse embryo.Development. 2015 May 15;142(10):1806-17. doi: 10.1242/dev.118927. Epub 2015 Apr 29. Development. 2015. PMID: 25926359 Free PMC article.
-
Persistence of histone H2AX phosphorylation after meiotic chromosome synapsis and abnormal centromere cohesion in poly (ADP-ribose) polymerase (Parp-1) null oocytes.Dev Biol. 2009 Jul 15;331(2):326-38. doi: 10.1016/j.ydbio.2009.05.550. Epub 2009 May 19. Dev Biol. 2009. PMID: 19463809 Free PMC article.
-
Role of ATRX in chromatin structure and function: implications for chromosome instability and human disease.Reproduction. 2011 Aug;142(2):221-34. doi: 10.1530/REP-10-0380. Epub 2011 Jun 8. Reproduction. 2011. PMID: 21653732 Free PMC article. Review.
Cited by
-
SWI/SNF chromatin remodeling subunit Smarca4/BRG1 is essential for female fertility†.Biol Reprod. 2023 Feb 13;108(2):279-291. doi: 10.1093/biolre/ioac209. Biol Reprod. 2023. PMID: 36440965 Free PMC article.
-
Atrx deficiency induces telomere dysfunction, endocrine defects, and reduced life span.J Clin Invest. 2013 May;123(5):2049-63. doi: 10.1172/JCI65634. Epub 2013 Apr 8. J Clin Invest. 2013. PMID: 23563309 Free PMC article.
-
The presence of the Y-chromosome, not the absence of the second X-chromosome, alters the mRNA levels stored in the fully grown XY mouse oocyte.PLoS One. 2012;7(7):e40481. doi: 10.1371/journal.pone.0040481. Epub 2012 Jul 6. PLoS One. 2012. PMID: 22792347 Free PMC article.
-
Chromatin remodeling of histone H3 variants underlies epigenetic inheritance of DNA methylation.bioRxiv [Preprint]. 2023 Aug 2:2023.07.11.548598. doi: 10.1101/2023.07.11.548598. bioRxiv. 2023. Update in: Cell. 2023 Sep 14;186(19):4100-4116.e15. doi: 10.1016/j.cell.2023.08.001 PMID: 37503143 Free PMC article. Updated. Preprint.
-
DAXX Is a Crucial Factor for Proper Development of Mammalian Oocytes and Early Embryos.Int J Mol Sci. 2021 Jan 28;22(3):1313. doi: 10.3390/ijms22031313. Int J Mol Sci. 2021. PMID: 33525665 Free PMC article. Review.
References
-
- Festenstein R, Sharghi-Namini S, Fox M, Roderick K, Tolaini M, et al. Heterochromatin protein 1 modifies mammalian PEV in a dose- and chromosomal-context-dependent manner. Nat Genet. 1999;23:457–461. - PubMed
-
- Bernard P, Maure J, Partridge J, Genier S, Javerzat J, et al. Requirement of heterochromatin for cohesion at centromeres. Science. 2001;294:2539–2542. - PubMed
-
- Bernard P, Allshire R. Centromeres become unstuck without heterochromatin. Trends Cell Biol. 2002;12:419–424. - PubMed
-
- Dillon N, Festenstein R. Unravelling heterochromatin: competition between positive and negative factors regulates accessibility. Trends Genet. 2002;18:252–258. - PubMed
-
- Cleveland D, Mao Y, Sullivan K. Centromeres and kinetochores: from epigenetics to mitotic checkpoint signaling. Cell. 2003;112:407–421. - PubMed
Publication types
MeSH terms
Substances
Grants and funding
LinkOut - more resources
Full Text Sources
Molecular Biology Databases