Fatty acid modulation and polyamine block of GluK2 kainate receptors analyzed by scanning mutagenesis
- PMID: 20805577
- PMCID: PMC2931155
- DOI: 10.1085/jgp.201010442
Fatty acid modulation and polyamine block of GluK2 kainate receptors analyzed by scanning mutagenesis
Abstract
RNA editing of kainate receptor subunits at the Q/R site determines their susceptibility to inhibition by cis-unsaturated fatty acids as well as block by cytoplasmic polyamines. Channels comprised of unedited (Q) subunits are strongly blocked by polyamines, but insensitive to fatty acids, such as arachidonic acid (AA) and docosahexaenoic acid (DHA), whereas homomeric edited (R) channels resist polyamine block but are inhibited by AA and DHA. In the present study, we have analyzed fatty acid modulation of whole-cell currents mediated by homomeric recombinant GluK2 (formerly GluR6) channels with individual residues in the pore-loop, M1 and M3 transmembrane helices replaced by scanning mutagenesis. Our results define three abutting surfaces along the M1, M2, and M3 helices where gain-of-function substitutions render GluK2(Q) channels susceptible to fatty acid inhibition. In addition, we identify four locations in the M3 helix (F611, L614, S618, and T621) at the level of the central cavity where Arg substitution increases relative permeability to chloride and eliminates polyamine block. Remarkably, for two of these positions, L614R and S618R, exposure to fatty acids reduces the apparent chloride permeability and potentiates whole-cell currents approximately 5 and 2.5-fold, respectively. Together, our results suggest that AA and DHA alter the orientation of M3 in the open state, depending on contacts at the interface between M1, M2, and M3. Moreover, our results demonstrate the importance of side chains within the central cavity in determining ionic selectivity and block by cytoplasmic polyamines despite the inverted orientation of GluK2 as compared with potassium channels and other pore-loop family members.
Figures
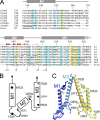
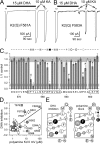
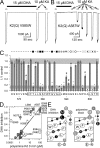
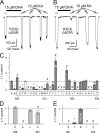
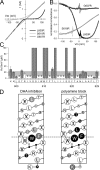
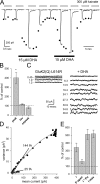
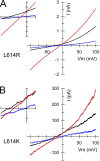
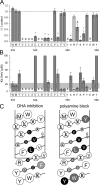
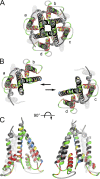
Similar articles
-
Amino acid substitutions in the pore helix of GluR6 control inhibition by membrane fatty acids.J Gen Physiol. 2008 Jul;132(1):85-99. doi: 10.1085/jgp.200810009. Epub 2008 Jun 18. J Gen Physiol. 2008. PMID: 18562501 Free PMC article.
-
Q/R site interactions with the M3 helix in GluK2 kainate receptor channels revealed by thermodynamic mutant cycles.J Gen Physiol. 2013 Sep;142(3):225-39. doi: 10.1085/jgp.201311000. Epub 2013 Aug 12. J Gen Physiol. 2013. PMID: 23940260 Free PMC article.
-
Structural similarities between glutamate receptor channels and K(+) channels examined by scanning mutagenesis.J Gen Physiol. 2001 Apr;117(4):345-60. doi: 10.1085/jgp.117.4.345. J Gen Physiol. 2001. PMID: 11279254 Free PMC article.
-
Genetic manipulation of key determinants of ion flow in glutamate receptor channels in the mouse.Brain Res. 2001 Jul 13;907(1-2):233-43. doi: 10.1016/s0006-8993(01)02445-3. Brain Res. 2001. PMID: 11430906 Review.
-
Emerging issues of connexin channels: biophysics fills the gap.Q Rev Biophys. 2001 Aug;34(3):325-472. doi: 10.1017/s0033583501003705. Q Rev Biophys. 2001. PMID: 11838236 Review.
Cited by
-
(n-3) fatty acids and cardiovascular health: are effects of EPA and DHA shared or complementary?J Nutr. 2012 Mar;142(3):614S-625S. doi: 10.3945/jn.111.149633. Epub 2012 Jan 25. J Nutr. 2012. PMID: 22279134 Free PMC article. Review.
-
Regulation of Ion Channel and Transporter Function Through RNA Editing.Curr Issues Mol Biol. 2015;17:23-36. Epub 2014 Oct 27. Curr Issues Mol Biol. 2015. PMID: 25347917 Free PMC article. Review.
-
Stargazin and cornichon-3 relieve polyamine block of AMPA receptors by enhancing blocker permeation.J Gen Physiol. 2018 Jan 2;150(1):67-82. doi: 10.1085/jgp.201711895. Epub 2017 Dec 8. J Gen Physiol. 2018. PMID: 29222130 Free PMC article.
-
Glutamate receptor pores.J Physiol. 2015 Jan 1;593(1):49-59. doi: 10.1113/jphysiol.2014.272724. Epub 2014 May 6. J Physiol. 2015. PMID: 25556787 Free PMC article. Review.
-
Omega-3 fatty acids lower blood pressure by directly activating large-conductance Ca²⁺-dependent K⁺ channels.Proc Natl Acad Sci U S A. 2013 Mar 19;110(12):4816-21. doi: 10.1073/pnas.1221997110. Epub 2013 Mar 4. Proc Natl Acad Sci U S A. 2013. PMID: 23487785 Free PMC article.
References
Publication types
MeSH terms
Substances
Grants and funding
LinkOut - more resources
Full Text Sources