Cysteine-based redox switches in enzymes
- PMID: 20799881
- PMCID: PMC3064533
- DOI: 10.1089/ars.2010.3376
Cysteine-based redox switches in enzymes
Abstract
The enzymes involved in metabolism and signaling are regulated by posttranslational modifications that influence their catalytic activity, rates of turnover, and targeting to subcellular locations. Most prominent among these has been phosphorylation/dephosphorylation, but now a distinct class of modification coming to the fore is a set of versatile redox modifications of key cysteine residues. Here we review the chemical, structural, and regulatory aspects of such redox regulation of enzymes and discuss examples of how these regulatory modifications often work in concert with phosphorylation/dephosphorylation events, making redox dependence an integral part of many cell signaling processes. Included are the emerging roles played by peroxiredoxins, a family of cysteine-based peroxidases that now appear to be major players in both antioxidant defense and cell signaling.
Figures
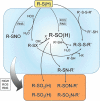
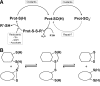
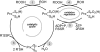
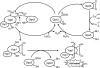
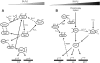
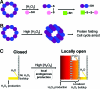
Similar articles
-
Depressing time: Waiting, melancholia, and the psychoanalytic practice of care.In: Kirtsoglou E, Simpson B, editors. The Time of Anthropology: Studies of Contemporary Chronopolitics. Abingdon: Routledge; 2020. Chapter 5. In: Kirtsoglou E, Simpson B, editors. The Time of Anthropology: Studies of Contemporary Chronopolitics. Abingdon: Routledge; 2020. Chapter 5. PMID: 36137063 Free Books & Documents. Review.
-
Qualitative evidence synthesis informing our understanding of people's perceptions and experiences of targeted digital communication.Cochrane Database Syst Rev. 2019 Oct 23;10(10):ED000141. doi: 10.1002/14651858.ED000141. Cochrane Database Syst Rev. 2019. PMID: 31643081 Free PMC article.
-
Australia in 2030: what is our path to health for all?Med J Aust. 2021 May;214 Suppl 8:S5-S40. doi: 10.5694/mja2.51020. Med J Aust. 2021. PMID: 33934362
-
Dynamic Field Theory of Executive Function: Identifying Early Neurocognitive Markers.Monogr Soc Res Child Dev. 2024 Dec;89(3):7-109. doi: 10.1111/mono.12478. Monogr Soc Res Child Dev. 2024. PMID: 39628288 Free PMC article.
-
Trends in Surgical and Nonsurgical Aesthetic Procedures: A 14-Year Analysis of the International Society of Aesthetic Plastic Surgery-ISAPS.Aesthetic Plast Surg. 2024 Oct;48(20):4217-4227. doi: 10.1007/s00266-024-04260-2. Epub 2024 Aug 5. Aesthetic Plast Surg. 2024. PMID: 39103642 Review.
Cited by
-
Protein thiol oxidation in the rat lung following e-cigarette exposure.Redox Biol. 2020 Oct;37:101758. doi: 10.1016/j.redox.2020.101758. Epub 2020 Oct 10. Redox Biol. 2020. PMID: 33080441 Free PMC article.
-
Selenite and tellurite form mixed seleno- and tellurotrisulfides with CstR from Staphylococcus aureus.Metallomics. 2013 Apr;5(4):335-42. doi: 10.1039/c3mt20205d. Metallomics. 2013. PMID: 23385876 Free PMC article.
-
Oxidative modification of proteins: an emerging mechanism of cell signaling.Front Physiol. 2012 Sep 14;3:369. doi: 10.3389/fphys.2012.00369. eCollection 2012. Front Physiol. 2012. PMID: 23049513 Free PMC article.
-
A deep redox proteome profiling workflow and its application to skeletal muscle of a Duchenne Muscular Dystrophy model.Free Radic Biol Med. 2022 Nov 20;193(Pt 1):373-384. doi: 10.1016/j.freeradbiomed.2022.10.300. Epub 2022 Oct 25. Free Radic Biol Med. 2022. PMID: 36306991 Free PMC article.
-
Changes in Free Radical Processes under the Influence of Low-Frequency Electromagnetic Field in Rats.Bull Exp Biol Med. 2022 Mar;172(5):566-569. doi: 10.1007/s10517-022-05434-1. Epub 2022 Mar 29. Bull Exp Biol Med. 2022. PMID: 35348955
References
-
- Allison WS. Formation and reactions of sulfenic acids in proteins. Acc Chem Res. 1976;9:293–299.
-
- Brown KK. Eriksson SE. Arner ES. Hampton MB. Mitochondrial peroxiredoxin 3 is rapidly oxidized in cells treated with isothiocyanates. Free Radic Biol Med. 2008;45:494–502. - PubMed
-
- Burgoyne JR. Madhani M. Cuello F. Charles RL. Brennan JP. Schroder E. Browning DD. Eaton P. Cysteine redox sensor in PKGIa enables oxidant-induced activation. Science. 2007;317:1393–1397. - PubMed
Publication types
MeSH terms
Substances
Grants and funding
LinkOut - more resources
Full Text Sources
Other Literature Sources