Brain glutamine synthesis requires neuronal-born aspartate as amino donor for glial glutamate formation
- PMID: 20736955
- PMCID: PMC3049464
- DOI: 10.1038/jcbfm.2010.146
Brain glutamine synthesis requires neuronal-born aspartate as amino donor for glial glutamate formation
Abstract
The glutamate-glutamine cycle faces a drain of glutamate by oxidation, which is balanced by the anaplerotic synthesis of glutamate and glutamine in astrocytes. De novo synthesis of glutamate by astrocytes requires an amino group whose origin is unknown. The deficiency in Aralar/AGC1, the main mitochondrial carrier for aspartate-glutamate expressed in brain, results in a drastic fall in brain glutamine production but a modest decrease in brain glutamate levels, which is not due to decreases in neuronal or synaptosomal glutamate content. In vivo (13)C nuclear magnetic resonance labeling with (13)C(2)acetate or (1-(13)C) glucose showed that the drop in brain glutamine is due to a failure in glial glutamate synthesis. Aralar deficiency induces a decrease in aspartate content, an increase in lactate production, and lactate-to-pyruvate ratio in cultured neurons but not in cultured astrocytes, indicating that Aralar is only functional in neurons. We find that aspartate, but not other amino acids, increases glutamate synthesis in both control and aralar-deficient astrocytes, mainly by serving as amino donor. These findings suggest the existence of a neuron-to-astrocyte aspartate transcellular pathway required for astrocyte glutamate synthesis and subsequent glutamine formation. This pathway may provide a mechanism to transfer neuronal-born redox equivalents to mitochondria in astrocytes.
Figures
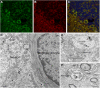
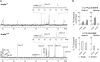
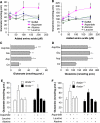
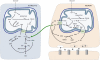
Comment in
-
Brain glutamine synthesis requires neuronal aspartate: a commentary.J Cereb Blood Flow Metab. 2011 Jan;31(1):384-7. doi: 10.1038/jcbfm.2010.199. Epub 2010 Nov 10. J Cereb Blood Flow Metab. 2011. PMID: 21063427 Free PMC article.
Similar articles
-
ARALAR/AGC1 deficiency, a neurodevelopmental disorder with severe impairment of neuronal mitochondrial respiration, does not produce a primary increase in brain lactate.J Neurochem. 2017 Jul;142(1):132-139. doi: 10.1111/jnc.14047. Epub 2017 May 22. J Neurochem. 2017. PMID: 28429368
-
Brain glutamine synthesis requires neuronal aspartate: a commentary.J Cereb Blood Flow Metab. 2011 Jan;31(1):384-7. doi: 10.1038/jcbfm.2010.199. Epub 2010 Nov 10. J Cereb Blood Flow Metab. 2011. PMID: 21063427 Free PMC article.
-
NMR spectroscopic study on the metabolic fate of [3-(13)C]alanine in astrocytes, neurons, and cocultures: implications for glia-neuron interactions in neurotransmitter metabolism.Glia. 2000 Dec;32(3):286-303. doi: 10.1002/1098-1136(200012)32:3<286::aid-glia80>3.0.co;2-p. Glia. 2000. PMID: 11102969
-
AGC1 Deficiency: Pathology and Molecular and Cellular Mechanisms of the Disease.Int J Mol Sci. 2022 Jan 4;23(1):528. doi: 10.3390/ijms23010528. Int J Mol Sci. 2022. PMID: 35008954 Free PMC article. Review.
-
Regulation of glial metabolism studied by 13C-NMR.NMR Biomed. 2003 Oct-Nov;16(6-7):370-99. doi: 10.1002/nbm.850. NMR Biomed. 2003. PMID: 14679501 Review.
Cited by
-
Deficient glucose and glutamine metabolism in Aralar/AGC1/Slc25a12 knockout mice contributes to altered visual function.Mol Vis. 2016 Oct 12;22:1198-1212. eCollection 2016. Mol Vis. 2016. PMID: 27746674 Free PMC article.
-
Multifactorial Effects on Different Types of Brain Cells Contribute to Ammonia Toxicity.Neurochem Res. 2017 Mar;42(3):721-736. doi: 10.1007/s11064-016-1966-1. Epub 2016 Jun 10. Neurochem Res. 2017. PMID: 27286679 Review.
-
Uncoupling Protein 2 (UCP2) Function in the Brain as Revealed by the Cerebral Metabolism of (1-13C)-Glucose.Neurochem Res. 2017 Jan;42(1):108-114. doi: 10.1007/s11064-016-1999-5. Epub 2016 Jul 12. Neurochem Res. 2017. PMID: 27401256
-
Ketogenic Diet Treatment of Defects in the Mitochondrial Malate Aspartate Shuttle and Pyruvate Carrier.Nutrients. 2022 Aug 31;14(17):3605. doi: 10.3390/nu14173605. Nutrients. 2022. PMID: 36079864 Free PMC article.
-
Non-targeted Metabolomics Profiling of Plasma Samples From Patients With Major Depressive Disorder.Front Psychiatry. 2022 Feb 21;12:810302. doi: 10.3389/fpsyt.2021.810302. eCollection 2021. Front Psychiatry. 2022. PMID: 35264984 Free PMC article.
References
-
- Aureli T, Di Cocco ME, Calvani M, Conti F. The entry of [1-13C]glucose into biochemical pathways reveals a complex compartimentation and metabolite trafficking between glia and neurons: a study by 13C-NMR spectroscopy. Brain Res. 1997;765:218–227. - PubMed
-
- Bak LK, Schousboe A, Waagepetersen HS. The glutamate/GABA-glutamine cycle: aspects of transport, neurotransmitter homeostasis and ammonia transfer. J Neurochem. 2006;98:641–653. - PubMed
-
- Berkich DA, Ola MS, Cole J, Sweatt AJ, Hutson SM, LaNoue KF. Mitochondrial transport proteins of the brain. J Neurosci Res. 2007;85:3367–3377. - PubMed
-
- Bröer S, Bröer A, Hansen JT, Bubb WA, Balcar VJ, Nasrallah FA, Garner B, Rae C. Alanine metabolism, transport, and cycling in the brain. J Neurochem. 2007;102:1758–1770. - PubMed
-
- Cahoy JD, Emery B, Kaushal A, Foo LC, Zamanian JL, Christopherson KS, Xing Y, Lubischer JL, Krieg PA, Krupenko SA, Thompson WJ, Barres BA. A transcriptome database for astrocytes, neurons, and oligodendrocytes: a new resource for understanding brain development and function. J Neurosci. 2008;28:264–278. - PMC - PubMed
Publication types
MeSH terms
Substances
LinkOut - more resources
Full Text Sources
Other Literature Sources