Precise spatiotemporal patterns among visual cortical areas and their relation to visual stimulus processing
- PMID: 20720131
- PMCID: PMC6633472
- DOI: 10.1523/JNEUROSCI.5177-09.2010
Precise spatiotemporal patterns among visual cortical areas and their relation to visual stimulus processing
Abstract
Visual processing shows a highly distributed organization in which the presentation of a visual stimulus simultaneously activates neurons in multiple columns across several cortical areas. It has been suggested that precise spatiotemporal activity patterns within and across cortical areas play a key role in higher cognitive, motor, and visual functions. In the visual system, these patterns have been proposed to take part in binding stimulus features into a coherent object, i.e., to be involved in perceptual grouping. Using voltage-sensitive dye imaging (VSDI) in behaving monkeys (Macaca fascicularis, males), we simultaneously measured neural population activity in the primary visual cortex (V1) and extrastriate cortex (V2, V4) at high spatial and temporal resolution. We detected time point population events (PEs) in the VSDI signal of each pixel and found that they reflect transient increased neural activation within local populations by establishing their relation to spiking and local field potential activity. Then, we searched for repeating space and time relations between the detected PEs. We demonstrate the following: (1) spatiotemporal patterns occurring within (horizontal) and across (vertical) early visual areas repeat significantly above chance level; (2) information carried in only a few patterns can be used to reliably discriminate between stimulus categories on a single-trial level; (3) the spatiotemporal patterns yielding high classification performance are characterized by late temporal occurrence and top-down propagation, which are consistent with cortical mechanisms involving perceptual grouping. The pattern characteristics and the robust relation between the patterns and the stimulus categories suggest that spatiotemporal activity patterns play an important role in cortical mechanisms of higher visual processing.
Figures
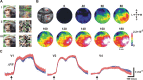
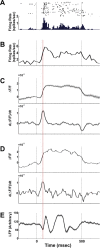
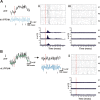
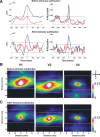
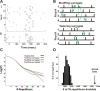
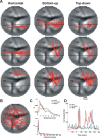
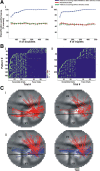
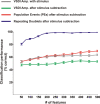
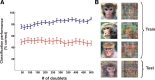
Similar articles
-
Long-term voltage-sensitive dye imaging reveals cortical dynamics in behaving monkeys.J Neurophysiol. 2002 Dec;88(6):3421-38. doi: 10.1152/jn.00194.2002. J Neurophysiol. 2002. PMID: 12466458
-
Population response to natural images in the primary visual cortex encodes local stimulus attributes and perceptual processing.J Neurosci. 2012 Oct 3;32(40):13971-86. doi: 10.1523/JNEUROSCI.1596-12.2012. J Neurosci. 2012. PMID: 23035105 Free PMC article.
-
Spatial and temporal scales of neuronal correlation in primary visual cortex.J Neurosci. 2008 Nov 26;28(48):12591-603. doi: 10.1523/JNEUROSCI.2929-08.2008. J Neurosci. 2008. PMID: 19036953 Free PMC article.
-
Imaging the Dynamics of Neocortical Population Activity in Behaving and Freely Moving Mammals.Adv Exp Med Biol. 2015;859:273-96. doi: 10.1007/978-3-319-17641-3_11. Adv Exp Med Biol. 2015. PMID: 26238057 Review.
-
Imaging the Dynamics of Mammalian Neocortical Population Activity In-Vivo.Adv Exp Med Biol. 2015;859:243-71. doi: 10.1007/978-3-319-17641-3_10. Adv Exp Med Biol. 2015. PMID: 26238056 Review.
Cited by
-
Local networks from different parts of the human cerebral cortex generate and share the same population dynamic.Cereb Cortex Commun. 2022 Oct 28;3(4):tgac040. doi: 10.1093/texcom/tgac040. eCollection 2022. Cereb Cortex Commun. 2022. PMID: 36530950 Free PMC article.
-
Consensus paper: pathological role of the cerebellum in autism.Cerebellum. 2012 Sep;11(3):777-807. doi: 10.1007/s12311-012-0355-9. Cerebellum. 2012. PMID: 22370873 Free PMC article. Review.
-
Neuronal ensembles: Building blocks of neural circuits.Neuron. 2024 Mar 20;112(6):875-892. doi: 10.1016/j.neuron.2023.12.008. Epub 2024 Jan 22. Neuron. 2024. PMID: 38262413 Review.
-
Endogenous sequential cortical activity evoked by visual stimuli.J Neurosci. 2015 Jun 10;35(23):8813-28. doi: 10.1523/JNEUROSCI.5214-14.2015. J Neurosci. 2015. PMID: 26063915 Free PMC article.
-
Classification of Spatiotemporal Neural Activity Patterns in Brain Imaging Data.Sci Rep. 2018 May 29;8(1):8231. doi: 10.1038/s41598-018-26605-z. Sci Rep. 2018. PMID: 29844346 Free PMC article.
References
-
- Abeles M. Studies of Brain Function. Berlin: Springer; 1982a. Local cortical circuits–an electrophysiological study; pp. 62–66.
-
- Abeles M. Role of the cortical neuron: integrator or coincidence detector? Isr J Med Sci. 1982b;18:83–92. - PubMed
-
- Abeles M. Corticonics: neural circuits of the cerebral cortex. New York: Cambridge UP; 1991.
-
- Abeles M, Bergman H, Margalit E, Vaadia E. Spatiotemporal firing patterns in the frontal cortex of behaving monkeys. J Neurophysiol. 1993;70:1629–1638. - PubMed
-
- Abeles M, Hayon G, Lehmann D. Modeling compositionality by dynamic binding of synfire chains. J Comput Neurosci. 2004;17:179–201. - PubMed
Publication types
MeSH terms
LinkOut - more resources
Full Text Sources