A Wnt-Frz/Ror-Dsh pathway regulates neurite outgrowth in Caenorhabditis elegans
- PMID: 20711352
- PMCID: PMC2920835
- DOI: 10.1371/journal.pgen.1001056
A Wnt-Frz/Ror-Dsh pathway regulates neurite outgrowth in Caenorhabditis elegans
Abstract
One of the challenges to understand the organization of the nervous system has been to determine how axon guidance molecules govern axon outgrowth. Through an unbiased genetic screen, we identified a conserved Wnt pathway which is crucial for anterior-posterior (A/P) outgrowth of neurites from RME head motor neurons in Caenorhabditis elegans. The pathway is composed of the Wnt ligand CWN-2, the Frizzled receptors CFZ-2 and MIG-1, the co-receptor CAM-1/Ror, and the downstream component Dishevelled/DSH-1. Among these, CWN-2 acts as a local attractive cue for neurite outgrowth, and its activity can be partially substituted with other Wnts, suggesting that spatial distribution plays a role in the functional specificity of Wnts. As a co-receptor, CAM-1 functions cell-autonomously in neurons and, together with CFZ-2 and MIG-1, transmits the Wnt signal to downstream effectors. Yeast two-hybrid screening identified DSH-1 as a binding partner for CAM-1, indicating that CAM-1 could facilitate CWN-2/Wnt signaling by its physical association with DSH-1. Our study reveals an important role of a Wnt-Frz/Ror-Dsh pathway in regulating neurite A/P outgrowth.
Conflict of interest statement
The authors have declared that no competing interests exist.
Figures
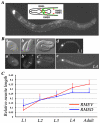
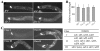
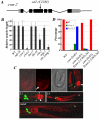
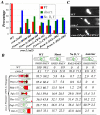
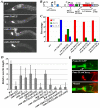
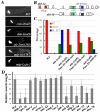
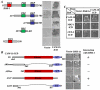
Similar articles
-
Robo and Ror function in a common receptor complex to regulate Wnt-mediated neurite outgrowth in Caenorhabditis elegans.Proc Natl Acad Sci U S A. 2018 Mar 6;115(10):E2254-E2263. doi: 10.1073/pnas.1717468115. Epub 2018 Feb 20. Proc Natl Acad Sci U S A. 2018. PMID: 29463707 Free PMC article.
-
Wnt-Ror signaling to SIA and SIB neurons directs anterior axon guidance and nerve ring placement in C. elegans.Development. 2009 Nov;136(22):3801-10. doi: 10.1242/dev.038109. Development. 2009. PMID: 19855022 Free PMC article.
-
Dishevelled attenuates the repelling activity of Wnt signaling during neurite outgrowth in Caenorhabditis elegans.Proc Natl Acad Sci U S A. 2015 Oct 27;112(43):13243-8. doi: 10.1073/pnas.1518686112. Epub 2015 Oct 12. Proc Natl Acad Sci U S A. 2015. PMID: 26460008 Free PMC article.
-
Ror receptor tyrosine kinases: orphans no more.Trends Cell Biol. 2008 Nov;18(11):536-44. doi: 10.1016/j.tcb.2008.08.006. Epub 2008 Oct 9. Trends Cell Biol. 2008. PMID: 18848778 Free PMC article. Review.
-
Should I stay or should I go: Wnt signals at the synapse.Cell. 2007 Aug 24;130(4):593-6. doi: 10.1016/j.cell.2007.08.007. Cell. 2007. PMID: 17719537 Review.
Cited by
-
Autonomous and nonautonomous regulation of Wnt-mediated neuronal polarity by the C. elegans Ror kinase CAM-1.Dev Biol. 2015 Aug 1;404(1):55-65. doi: 10.1016/j.ydbio.2015.04.015. Epub 2015 Apr 24. Dev Biol. 2015. PMID: 25917219 Free PMC article.
-
The role of Ryk and Ror receptor tyrosine kinases in Wnt signal transduction.Cold Spring Harb Perspect Biol. 2014 Feb 1;6(2):a009175. doi: 10.1101/cshperspect.a009175. Cold Spring Harb Perspect Biol. 2014. PMID: 24370848 Free PMC article. Review.
-
A CRISPR Tagging-Based Screen Reveals Localized Players in Wnt-Directed Asymmetric Cell Division.Genetics. 2018 Mar;208(3):1147-1164. doi: 10.1534/genetics.117.300487. Epub 2018 Jan 18. Genetics. 2018. PMID: 29348144 Free PMC article.
-
Synaptotagmin 1 directs repetitive release by coupling vesicle exocytosis to the Rab3 cycle.Elife. 2015 Feb 24;4:e05118. doi: 10.7554/eLife.05118. Elife. 2015. PMID: 25710274 Free PMC article.
-
Intersection of AHR and Wnt signaling in development, health, and disease.Int J Mol Sci. 2014 Oct 3;15(10):17852-85. doi: 10.3390/ijms151017852. Int J Mol Sci. 2014. PMID: 25286307 Free PMC article. Review.
References
-
- Tessier-Lavigne M, Goodman CS. The molecular biology of axon guidance. Science. 1996;274:1123–1133. - PubMed
-
- Dickson BJ. Molecular mechanisms of axon guidance. Science. 2002;298:1959–1964. - PubMed
-
- Yu TW, Bargmann CI. Dynamic regulation of axon guidance. Nat Neurosci. 2001;4(Suppl):1169–1176. - PubMed
-
- Huber AB, Kolodkin AL, Ginty DD, Cloutier JF. Signaling at the growth cone: ligand-receptor complexes and the control of axon growth and guidance. Annu Rev Neurosci. 2003;26:509–563. - PubMed
-
- Zou Y. Wnt signaling in axon guidance. Trends Neurosci. 2004;27:528–532. - PubMed
Publication types
MeSH terms
Substances
LinkOut - more resources
Full Text Sources
Molecular Biology Databases
Research Materials