Stretchy proteins on stretchy substrates: the important elements of integrin-mediated rigidity sensing
- PMID: 20708583
- PMCID: PMC5319208
- DOI: 10.1016/j.devcel.2010.07.018
Stretchy proteins on stretchy substrates: the important elements of integrin-mediated rigidity sensing
Abstract
Matrix and tissue rigidity guides many cellular processes, including the differentiation of stem cells and the migration of cells in health and disease. Cells actively and transiently test rigidity using mechanisms limited by inherent physical parameters that include the strength of extracellular attachments, the pulling capacity on these attachments, and the sensitivity of the mechanotransduction system. Here, we focus on rigidity sensing mediated through the integrin family of extracellular matrix receptors and linked proteins and discuss the evidence supporting these proteins as mechanosensors.
2010 Elsevier Inc. All rights reserved.
Figures
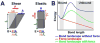
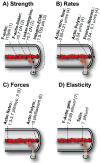
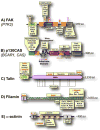
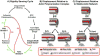
Similar articles
-
Integrin diversity brings specificity in mechanotransduction.Biol Cell. 2018 Mar;110(3):49-64. doi: 10.1111/boc.201700060. Epub 2018 Feb 15. Biol Cell. 2018. PMID: 29388220 Review.
-
Integrin-mediated adhesion and mechanosensing in the mammary gland.Semin Cell Dev Biol. 2021 Jun;114:113-125. doi: 10.1016/j.semcdb.2020.10.010. Epub 2020 Nov 10. Semin Cell Dev Biol. 2021. PMID: 33187835
-
Focal adhesions as mechanosensors: the two-spring model.Biosystems. 2006 Feb-Mar;83(2-3):225-32. doi: 10.1016/j.biosystems.2005.05.019. Epub 2005 Oct 19. Biosystems. 2006. PMID: 16236431
-
Regulation of matrix assembly through rigidity-dependent fibronectin conformational changes.J Biol Chem. 2013 May 24;288(21):14805-14. doi: 10.1074/jbc.M112.435271. Epub 2013 Apr 15. J Biol Chem. 2013. PMID: 23589296 Free PMC article.
-
Dynamic regulation of the structure and functions of integrin adhesions.Dev Cell. 2013 Mar 11;24(5):447-58. doi: 10.1016/j.devcel.2013.02.012. Dev Cell. 2013. PMID: 23484852 Free PMC article. Review.
Cited by
-
Matrix, mesenchyme, and mechanotransduction.Ann Am Thorac Soc. 2015 Mar;12 Suppl 1(Suppl 1):S24-9. doi: 10.1513/AnnalsATS.201407-320MG. Ann Am Thorac Soc. 2015. PMID: 25830830 Free PMC article. Review.
-
A plastic relationship between vinculin-mediated tension and adhesion complex area defines adhesion size and lifetime.Nat Commun. 2015 Jun 25;6:7524. doi: 10.1038/ncomms8524. Nat Commun. 2015. PMID: 26109125 Free PMC article.
-
Vinculin-dependent Cadherin mechanosensing regulates efficient epithelial barrier formation.Biol Open. 2012 Nov 15;1(11):1128-40. doi: 10.1242/bio.20122428. Epub 2012 Sep 11. Biol Open. 2012. PMID: 23213393 Free PMC article.
-
Strength in the periphery: growth cone biomechanics and substrate rigidity response in peripheral and central nervous system neurons.Biophys J. 2012 Feb 8;102(3):452-60. doi: 10.1016/j.bpj.2011.12.025. Epub 2012 Feb 7. Biophys J. 2012. PMID: 22325267 Free PMC article.
-
Environmental Elasticity Regulates Cell-type Specific RHOA Signaling and Neuritogenesis of Human Neurons.Stem Cell Reports. 2019 Dec 10;13(6):1006-1021. doi: 10.1016/j.stemcr.2019.10.008. Epub 2019 Nov 7. Stem Cell Reports. 2019. PMID: 31708476 Free PMC article.
References
-
- Ando J, Yamamoto K. Vascular mechanobiology: endothelial cell responses to fluid shear stress. Circ J. 2009;73:1983–92. - PubMed
-
- Arnold M, Cavalcanti-Adam EA, Glass R, Blummel J, Eck W, Kantlehner M, Kessler H, Spatz JP. Activation of integrin function by nanopatterned adhesive interfaces. Chemphyschem. 2004;5:383–8. - PubMed
-
- Balaban NQ, Schwarz US, Riveline D, Goichberg P, Tzur G, Sabanay I, Mahalu D, Safran S, Bershadsky A, Addadi L, Geiger B. Force and focal adhesion assembly: a close relationship studied using elastic micropatterned substrates. Nat Cell Biol. 2001;3:466–72. - PubMed
-
- Balgude AP, Yu X, Szymanski A, Bellamkonda RV. Agarose gel stiffness determines rate of DRG neurite extension in 3D cultures. Biomaterials. 2001;22:1077–84. - PubMed
Publication types
MeSH terms
Substances
Grants and funding
LinkOut - more resources
Full Text Sources
Other Literature Sources