Palmitoylation of the S0-S1 linker regulates cell surface expression of voltage- and calcium-activated potassium (BK) channels
- PMID: 20693285
- PMCID: PMC2963414
- DOI: 10.1074/jbc.M110.153940
Palmitoylation of the S0-S1 linker regulates cell surface expression of voltage- and calcium-activated potassium (BK) channels
Abstract
S-palmitoylation is rapidly emerging as an important post-translational mechanism to regulate ion channels. We have previously demonstrated that large conductance calcium- and voltage-activated potassium (BK) channels are palmitoylated within an alternatively spliced (STREX) insert. However, these studies also revealed that additional site(s) for palmitoylation must exist outside of the STREX insert, although the identity or the functional significance of these palmitoylated cysteine residues are unknown. Here, we demonstrate that BK channels are palmitoylated at a cluster of evolutionary conserved cysteine residues (Cys-53, Cys-54, and Cys-56) within the intracellular linker between the S0 and S1 transmembrane domains. Mutation of Cys-53, Cys-54, and Cys-56 completely abolished palmitoylation of BK channels lacking the STREX insert (ZERO variant). Palmitoylation allows the S0-S1 linker to associate with the plasma membrane but has no effect on single channel conductance or the calcium/voltage sensitivity. Rather, S0-S1 linker palmitoylation is a critical determinant of cell surface expression of BK channels, as steady state surface expression levels are reduced by ∼55% in the C53:54:56A mutant. STREX variant channels that could not be palmitoylated in the S0-S1 linker also displayed significantly reduced cell surface expression even though STREX insert palmitoylation was unaffected. Thus our work reveals the functional independence of two distinct palmitoylation-dependent membrane interaction domains within the same channel protein and demonstrates the critical role of S0-S1 linker palmitoylation in the control of BK channel cell surface expression.
Figures
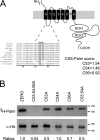
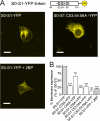
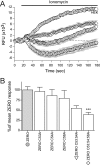
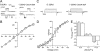
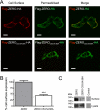
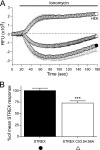
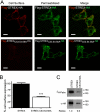
Similar articles
-
Palmitoylation gates phosphorylation-dependent regulation of BK potassium channels.Proc Natl Acad Sci U S A. 2008 Dec 30;105(52):21006-11. doi: 10.1073/pnas.0806700106. Epub 2008 Dec 19. Proc Natl Acad Sci U S A. 2008. PMID: 19098106 Free PMC article.
-
Multiple palmitoyltransferases are required for palmitoylation-dependent regulation of large conductance calcium- and voltage-activated potassium channels.J Biol Chem. 2010 Jul 30;285(31):23954-62. doi: 10.1074/jbc.M110.137802. Epub 2010 May 27. J Biol Chem. 2010. PMID: 20507996 Free PMC article.
-
Palmitoylation of the β4-subunit regulates surface expression of large conductance calcium-activated potassium channel splice variants.J Biol Chem. 2013 May 3;288(18):13136-44. doi: 10.1074/jbc.M113.461830. Epub 2013 Mar 16. J Biol Chem. 2013. PMID: 23504458 Free PMC article.
-
Distinct post-translational modifications regulate BK channel activity: the interplay between protein palmitoylation and phosphorylation.Channels (Austin). 2009 May-Jun;3(3):144-5. doi: 10.4161/chan.3.3.9257. Epub 2009 May 12. Channels (Austin). 2009. PMID: 19556862 Review. No abstract available.
-
Posttranscriptional and Posttranslational Regulation of BK Channels.Int Rev Neurobiol. 2016;128:91-126. doi: 10.1016/bs.irn.2016.02.012. Epub 2016 Mar 3. Int Rev Neurobiol. 2016. PMID: 27238262 Review.
Cited by
-
A BK (Slo1) channel journey from molecule to physiology.Channels (Austin). 2013 Nov-Dec;7(6):442-58. doi: 10.4161/chan.26242. Epub 2013 Sep 11. Channels (Austin). 2013. PMID: 24025517 Free PMC article. Review.
-
Site-specific deacylation by ABHD17a controls BK channel splice variant activity.J Biol Chem. 2020 Dec 4;295(49):16487-16496. doi: 10.1074/jbc.RA120.015349. Epub 2020 Sep 10. J Biol Chem. 2020. PMID: 32913120 Free PMC article.
-
Distinct acyl protein transferases and thioesterases control surface expression of calcium-activated potassium channels.J Biol Chem. 2012 Apr 27;287(18):14718-25. doi: 10.1074/jbc.M111.335547. Epub 2012 Mar 7. J Biol Chem. 2012. PMID: 22399288 Free PMC article.
-
Palmitoylation of Voltage-Gated Ion Channels.Int J Mol Sci. 2022 Aug 19;23(16):9357. doi: 10.3390/ijms23169357. Int J Mol Sci. 2022. PMID: 36012639 Free PMC article. Review.
-
BK channel activators and their therapeutic perspectives.Front Physiol. 2014 Oct 9;5:389. doi: 10.3389/fphys.2014.00389. eCollection 2014. Front Physiol. 2014. PMID: 25346695 Free PMC article. Review.
References
-
- Brenner R., Peréz G. J., Bonev A. D., Eckman D. M., Kosek J. C., Wiler S. W., Patterson A. J., Nelson M. T., Aldrich R. W. (2000) Nature 407, 870–876 - PubMed
-
- Sausbier M., Arntz C., Bucurenciu I., Zhao H., Zhou X. B., Sausbier U., Feil S., Kamm S., Essin K., Sailer C. A., Abdullah U., Krippeit-Drews P., Feil R., Hofmann F., Knaus H. G., Kenyon C., Shipston M. J., Storm J. F., Neuhuber W., Korth M., Schubert R., Gollasch M., Ruth P. (2005) Circulation 112, 60–68 - PubMed
-
- Brenner R., Chen Q. H., Vilaythong A., Toney G. M., Noebels J. L., Aldrich R. W. (2005) Nat. Neurosci. 8, 1752–1759 - PubMed
Publication types
MeSH terms
Substances
Grants and funding
LinkOut - more resources
Full Text Sources
Molecular Biology Databases