Drosophila PAT1 is required for Kinesin-1 to transport cargo and to maximize its motility
- PMID: 20630947
- PMCID: PMC2910386
- DOI: 10.1242/dev.048108
Drosophila PAT1 is required for Kinesin-1 to transport cargo and to maximize its motility
Abstract
Kinesin heavy chain (KHC), the force-generating component of Kinesin-1, is required for the localization of oskar mRNA and the anchoring of the nucleus in the Drosophila oocyte. These events are crucial for the establishment of the anterior-posterior and dorsal-ventral axes. KHC is also essential for the localization of Dynein and for all ooplasmic flows. Interestingly, oocytes without Kinesin light chain show no major defects in these KHC-dependent processes, suggesting that KHC binds its cargoes and is activated by a novel mechanism. Here, we shed new light on the molecular mechanism of Kinesin function in the germline. Using a combination of genetic, biochemical and motor-tracking studies, we show that PAT1, an APP-binding protein, interacts with Kinesin-1, functions in the transport of oskar mRNA and Dynein and is required for the efficient motility of KHC along microtubules. This work suggests that the role of PAT1 in cargo transport in the cell is linked to PAT1 function as a positive regulator of Kinesin motility.
Figures
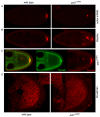
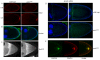
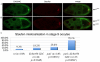
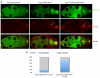
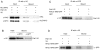
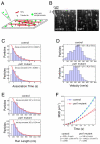
Similar articles
-
Kinesin light chain-independent function of the Kinesin heavy chain in cytoplasmic streaming and posterior localisation in the Drosophila oocyte.Development. 2002 Dec;129(23):5473-85. doi: 10.1242/dev.00119. Development. 2002. PMID: 12403717
-
A new isoform of Drosophila non-muscle Tropomyosin 1 interacts with Kinesin-1 and functions in oskar mRNA localization.J Cell Sci. 2016 Nov 15;129(22):4252-4264. doi: 10.1242/jcs.194332. Epub 2016 Oct 17. J Cell Sci. 2016. PMID: 27802167 Free PMC article.
-
Posterior localization of dynein and dorsal-ventral axis formation depend on kinesin in Drosophila oocytes.Curr Biol. 2002 Sep 3;12(17):1541-5. doi: 10.1016/s0960-9822(02)01108-9. Curr Biol. 2002. PMID: 12225672 Free PMC article.
-
Role of kinesin-1-based microtubule sliding in Drosophila nervous system development.Proc Natl Acad Sci U S A. 2016 Aug 23;113(34):E4985-94. doi: 10.1073/pnas.1522416113. Epub 2016 Aug 10. Proc Natl Acad Sci U S A. 2016. PMID: 27512046 Free PMC article.
-
Kinesin-1 captures RNA cargo in its adaptable coils.Genes Dev. 2021 Jul 1;35(13-14):937-939. doi: 10.1101/gad.348691.121. Genes Dev. 2021. PMID: 34210804 Free PMC article. Review.
Cited by
-
Molecular motors: directing traffic during RNA localization.Crit Rev Biochem Mol Biol. 2011 Jun;46(3):229-39. doi: 10.3109/10409238.2011.572861. Epub 2011 Apr 11. Crit Rev Biochem Mol Biol. 2011. PMID: 21476929 Free PMC article. Review.
-
A kinesin adapter directly mediates dendritic mRNA localization during neural development in mice.J Biol Chem. 2020 May 8;295(19):6605-6628. doi: 10.1074/jbc.RA118.005616. Epub 2020 Feb 28. J Biol Chem. 2020. PMID: 32111743 Free PMC article.
-
Cytoplasmic streaming in Drosophila oocytes varies with kinesin activity and correlates with the microtubule cytoskeleton architecture.Proc Natl Acad Sci U S A. 2012 Sep 18;109(38):15109-14. doi: 10.1073/pnas.1203575109. Epub 2012 Sep 4. Proc Natl Acad Sci U S A. 2012. PMID: 22949706 Free PMC article.
-
Klar ensures thermal robustness of oskar localization by restraining RNP motility.J Cell Biol. 2014 Jul 21;206(2):199-215. doi: 10.1083/jcb.201310010. J Cell Biol. 2014. PMID: 25049271 Free PMC article.
-
An RNA-binding atypical tropomyosin recruits kinesin-1 dynamically to oskar mRNPs.EMBO J. 2017 Feb 1;36(3):319-333. doi: 10.15252/embj.201696038. Epub 2016 Dec 27. EMBO J. 2017. PMID: 28028052 Free PMC article.
References
-
- Bowman A. B., Kamal A., Ritchings B. W., Philp A. V., McGrail M., Gindhart J. G., Goldstein L. S. (2000). Kinesin-dependent axonal transport is mediated by the sunday driver (SYD) protein. Cell 103, 583-594 - PubMed
Publication types
MeSH terms
Substances
Grants and funding
LinkOut - more resources
Full Text Sources
Molecular Biology Databases
Miscellaneous