Systems-level metabolic flux profiling elucidates a complete, bifurcated tricarboxylic acid cycle in Clostridium acetobutylicum
- PMID: 20622067
- PMCID: PMC2937365
- DOI: 10.1128/JB.00490-10
Systems-level metabolic flux profiling elucidates a complete, bifurcated tricarboxylic acid cycle in Clostridium acetobutylicum
Erratum in
- J Bacteriol. 2011 Dec;193(23):6805
Abstract
Obligatory anaerobic bacteria are major contributors to the overall metabolism of soil and the human gut. The metabolic pathways of these bacteria remain, however, poorly understood. Using isotope tracers, mass spectrometry, and quantitative flux modeling, here we directly map the metabolic pathways of Clostridium acetobutylicum, a soil bacterium whose major fermentation products include the biofuels butanol and hydrogen. While genome annotation suggests the absence of most tricarboxylic acid (TCA) cycle enzymes, our results demonstrate that this bacterium has a complete, albeit bifurcated, TCA cycle; oxaloacetate flows to succinate both through citrate/alpha-ketoglutarate and via malate/fumarate. Our investigations also yielded insights into the pathways utilized for glucose catabolism and amino acid biosynthesis and revealed that the organism's one-carbon metabolism is distinct from that of model microbes, involving reversible pyruvate decarboxylation and the use of pyruvate as the one-carbon donor for biosynthetic reactions. This study represents the first in vivo characterization of the TCA cycle and central metabolism of C. acetobutylicum. Our results establish a role for the full TCA cycle in an obligatory anaerobic organism and demonstrate the importance of complementing genome annotation with isotope tracer studies for determining the metabolic pathways of diverse microbes.
Figures
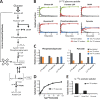
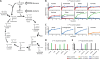
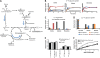
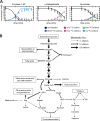
Similar articles
-
Resolving the TCA cycle and pentose-phosphate pathway of Clostridium acetobutylicum ATCC 824: Isotopomer analysis, in vitro activities and expression analysis.Biotechnol J. 2011 Mar;6(3):300-5. doi: 10.1002/biot.201000282. Epub 2010 Nov 4. Biotechnol J. 2011. PMID: 21370473 Free PMC article.
-
Parallel labeling experiments validate Clostridium acetobutylicum metabolic network model for (13)C metabolic flux analysis.Metab Eng. 2014 Nov;26:23-33. doi: 10.1016/j.ymben.2014.08.002. Epub 2014 Aug 23. Metab Eng. 2014. PMID: 25183671
-
Metabolome remodeling during the acidogenic-solventogenic transition in Clostridium acetobutylicum.Appl Environ Microbiol. 2011 Nov;77(22):7984-97. doi: 10.1128/AEM.05374-11. Epub 2011 Sep 23. Appl Environ Microbiol. 2011. PMID: 21948824 Free PMC article.
-
Metabolic engineering of Clostridium acetobutylicum: recent advances to improve butanol production.Curr Opin Biotechnol. 2011 Oct;22(5):634-47. doi: 10.1016/j.copbio.2011.01.011. Epub 2011 Mar 4. Curr Opin Biotechnol. 2011. PMID: 21377350 Review.
-
Application of new metabolic engineering tools for Clostridium acetobutylicum.Appl Microbiol Biotechnol. 2014 Jul;98(13):5823-37. doi: 10.1007/s00253-014-5785-5. Epub 2014 May 10. Appl Microbiol Biotechnol. 2014. PMID: 24816621 Review.
Cited by
-
Phosphoketolase pathway for xylose catabolism in Clostridium acetobutylicum revealed by 13C metabolic flux analysis.J Bacteriol. 2012 Oct;194(19):5413-22. doi: 10.1128/JB.00713-12. Epub 2012 Aug 3. J Bacteriol. 2012. PMID: 22865845 Free PMC article.
-
Phylogenomic analysis and predicted physiological role of the proton-translocating NADH:quinone oxidoreductase (complex I) across bacteria.mBio. 2015 Apr 14;6(2):e00389-15. doi: 10.1128/mBio.00389-15. mBio. 2015. PMID: 25873378 Free PMC article.
-
13C-metabolic flux analysis reveals metabolic rewiring in HL-60 neutrophil-like cells through differentiation and immune stimulation.Metab Eng Commun. 2024 May 27;18:e00239. doi: 10.1016/j.mec.2024.e00239. eCollection 2024 Jun. Metab Eng Commun. 2024. PMID: 38883865 Free PMC article.
-
A universal mariner transposon system for forward genetic studies in the genus Clostridium.PLoS One. 2015 Apr 2;10(4):e0122411. doi: 10.1371/journal.pone.0122411. eCollection 2015. PLoS One. 2015. PMID: 25836262 Free PMC article.
-
Glucose transporter 1 is important for the glycolytic metabolism of human endometrial stromal cells in hypoxic environment.Heliyon. 2020 Jun 8;6(6):e03985. doi: 10.1016/j.heliyon.2020.e03985. eCollection 2020 Jun. Heliyon. 2020. PMID: 32548315 Free PMC article.
References
-
- Bajad, S. U., W. Lu, E. H. Kimball, J. Yuan, C. Peterson, and J. D. Rabinowitz. 2006. Separation and quantitation of water soluble cellular metabolites by hydrophilic interaction chromatography-tandem mass spectrometry. J. Chromatogr. A 1125:76-88. - PubMed
-
- Brinkac, L. M., T. Davidsen, E. Beck, A. Ganapathy, E. Caler, R. J. Dodson, A. S. Durkin, D. M. Harkins, H. Lorenzi, R. Madupu, Y. Sebastian, S. Shrivastava, M. Thiagarajan, J. Orvis, J. P. Sundaram, J. Crabtree, K. Galens, Y. Zhao, J. M. Inman, R. Montgomery, S. Schobel, K. Galinsky, D. M. Tanenbaum, A. Resnick, N. Zafar, O. White, and G. Sutton. 2010. Pathema: a clade-specific bioinformatics resource center for pathogen research. Nucleic Acids Res. 38:D408-D414. - PMC - PubMed
-
- Dehal, P. S., M. P. Joachimiak, M. N. Price, J. T. Bates, J. K. Baumohl, D. Chivian, G. D. Friedland, K. H. Huang, K. Keller, P. S. Novichkov, I. L. Dubchak, E. J. Alm, and A. P. Arkin. 2010. MicrobesOnline: an integrated portal for comparative and functional genomics. Nucleic Acids Res. 38:D396-D400. - PMC - PubMed
-
- Dürre, P. 2005. Handbook on clostridia. Taylor & Francis, Boca Raton, FL.
MeSH terms
Substances
LinkOut - more resources
Full Text Sources
Other Literature Sources