Contribution of the S5-pore-S6 domain to the gating characteristics of the cation channels TRPM2 and TRPM8
- PMID: 20587417
- PMCID: PMC2930679
- DOI: 10.1074/jbc.M110.109975
Contribution of the S5-pore-S6 domain to the gating characteristics of the cation channels TRPM2 and TRPM8
Abstract
The closely related cation channels TRPM2 and TRPM8 show completely different requirements for stimulation and are regulated by Ca(2+) in an opposite manner. TRPM8 is basically gated in a voltage-dependent process enhanced by cold temperatures and cooling compounds such as menthol and icilin. The putative S4 voltage sensor of TRPM8 is closely similar to that of TRPM2, which, however, is mostly devoid of voltage sensitivity. To gain insight into principal interactions of critical channel domains during the gating process, we created chimeras in which the entire S5-pore-S6 domains were reciprocally exchanged. The chimera M2-M8P (i.e. TRPM2 with the pore of TRPM8) responded to ADP-ribose and hydrogen peroxide and was regulated by extracellular and intracellular Ca(2+) as was wild-type TRPM2. Single-channel recordings revealed the characteristic pattern of TRPM2 with extremely long open times. Only at far-negative membrane potentials (-120 to -140 mV) did differences become apparent because currents were reduced by hyperpolarization in M2-M8P but not in TRPM2. The reciprocal chimera, M8-M2P, showed currents after stimulation with high concentrations of menthol and icilin, but these currents were only slightly larger than in controls. The transfer of the NUDT9 domain to the C terminus of TRPM8 produced a channel sensitive to cold, menthol, or icilin but insensitive to ADP-ribose or hydrogen peroxide. We conclude that the gating processes in TRPM2 and TRPM8 differ in their requirements for specific structures within the pore. Moreover, the regulation by extracellular and intracellular Ca(2+) and the single-channel properties in TRPM2 are not determined by the S5-pore-S6 region.
Figures
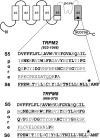
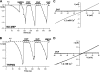
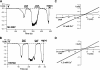
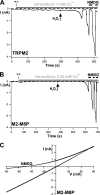
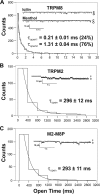
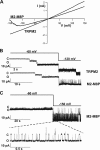
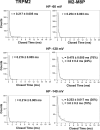
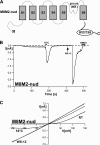
Similar articles
-
Inhibition of TRPM8 by icilin distinct from desensitization induced by menthol and menthol derivatives.J Biol Chem. 2009 Feb 13;284(7):4102-11. doi: 10.1074/jbc.M806651200. Epub 2008 Dec 18. J Biol Chem. 2009. PMID: 19095656
-
Importance of a conserved sequence motif in transmembrane segment S3 for the gating of human TRPM8 and TRPM2.PLoS One. 2012;7(11):e49877. doi: 10.1371/journal.pone.0049877. Epub 2012 Nov 21. PLoS One. 2012. PMID: 23185472 Free PMC article.
-
The transmembrane segment S6 determines cation versus anion selectivity of TRPM2 and TRPM8.J Biol Chem. 2007 Sep 21;282(38):27598-609. doi: 10.1074/jbc.M702247200. Epub 2007 Jun 29. J Biol Chem. 2007. PMID: 17604279
-
Regulation of TRPM8 channel activity.Mol Cell Endocrinol. 2012 Apr 28;353(1-2):68-74. doi: 10.1016/j.mce.2011.10.023. Epub 2011 Oct 28. Mol Cell Endocrinol. 2012. PMID: 22061619 Free PMC article. Review.
-
Mechanism of TRPM2 channel gating revealed by cryo-EM.FEBS J. 2019 Sep;286(17):3333-3339. doi: 10.1111/febs.14939. Epub 2019 Jun 10. FEBS J. 2019. PMID: 31144442 Free PMC article. Review.
Cited by
-
ADP-Ribose Activates the TRPM2 Channel from the Sea Anemone Nematostella vectensis Independently of the NUDT9H Domain.PLoS One. 2016 Jun 22;11(6):e0158060. doi: 10.1371/journal.pone.0158060. eCollection 2016. PLoS One. 2016. PMID: 27333281 Free PMC article.
-
Different Principles of ADP-Ribose-Mediated Activation and Opposite Roles of the NUDT9 Homology Domain in the TRPM2 Orthologs of Man and Sea Anemone.Front Physiol. 2017 Oct 31;8:879. doi: 10.3389/fphys.2017.00879. eCollection 2017. Front Physiol. 2017. PMID: 29163217 Free PMC article. Review.
-
Current View of Ligand and Lipid Recognition by the Menthol Receptor TRPM8.Trends Biochem Sci. 2020 Sep;45(9):806-819. doi: 10.1016/j.tibs.2020.05.008. Epub 2020 Jun 9. Trends Biochem Sci. 2020. PMID: 32532587 Free PMC article. Review.
-
Structure-Function Relationship of TRPM2: Recent Advances, Contradictions, and Open Questions.Int J Mol Sci. 2020 Sep 4;21(18):6481. doi: 10.3390/ijms21186481. Int J Mol Sci. 2020. PMID: 32899872 Free PMC article. Review.
-
Drosophila menthol sensitivity and the Precambrian origins of transient receptor potential-dependent chemosensation.Philos Trans R Soc Lond B Biol Sci. 2019 Nov 11;374(1785):20190369. doi: 10.1098/rstb.2019.0369. Epub 2019 Sep 23. Philos Trans R Soc Lond B Biol Sci. 2019. PMID: 31544603 Free PMC article.
References
-
- Peier A. M., Moqrich A., Hergarden A. C., Reeve A. J., Andersson D. A., Story G. M., Earley T. J., Dragoni I., McIntyre P., Bevan S., Patapoutian A. (2002) Cell 108, 705–715 - PubMed
-
- Perraud A. L., Fleig A., Dunn C. A., Bagley L. A., Launay P., Schmitz C., Stokes A. J., Zhu Q., Bessman M. J., Penner R., Kinet J. P., Scharenberg A. M. (2001) Nature 411, 595–599 - PubMed
-
- Hara Y., Wakamori M., Ishii M., Maeno E., Nishida M., Yoshida T., Yamada H., Shimizu S., Mori E., Kudoh J., Shimizu N., Kurose H., Okada Y., Imoto K., Mori Y. (2002) Mol. Cell 9, 163–173 - PubMed
-
- Kühn F. J., Lückhoff A. (2004) J. Biol. Chem. 279, 46431–46437 - PubMed
-
- Perraud A. L., Takanishi C. L., Shen B., Kang S., Smith M. K., Schmitz C., Knowles H. M., Ferraris D., Li W., Zhang J., Stoddard B. L., Scharenberg A. M. (2005) J. Biol. Chem. 280, 6138–6148 - PubMed
Publication types
MeSH terms
Substances
LinkOut - more resources
Full Text Sources
Research Materials
Miscellaneous