Regulation of the cardiomyocyte transcriptome vs translatome by endothelin-1 and insulin: translational regulation of 5' terminal oligopyrimidine tract (TOP) mRNAs by insulin
- PMID: 20509958
- PMCID: PMC2900265
- DOI: 10.1186/1471-2164-11-343
Regulation of the cardiomyocyte transcriptome vs translatome by endothelin-1 and insulin: translational regulation of 5' terminal oligopyrimidine tract (TOP) mRNAs by insulin
Abstract
Background: Changes in cellular phenotype result from underlying changes in mRNA transcription and translation. Endothelin-1 stimulates cardiomyocyte hypertrophy with associated changes in mRNA/protein expression and an increase in the rate of protein synthesis. Insulin also increases the rate of translation but does not promote overt cardiomyocyte hypertrophy. One mechanism of translational regulation is through 5' terminal oligopyrimidine tracts (TOPs) that, in response to growth stimuli, promote mRNA recruitment to polysomes for increased translation. TOP mRNAs include those encoding ribosomal proteins, but the full panoply remains to be established. Here, we used microarrays to compare the effects of endothelin-1 and insulin on the global transcriptome of neonatal rat cardiomyocytes, and on mRNA recruitment to polysomes (i.e. the translatome).
Results: Globally, endothelin-1 and insulin (1 h) promoted >1.5-fold significant (false discovery rate < 0.05) changes in expression of 341 and 38 RNAs, respectively. For these transcripts with this level of change there was little evidence of translational regulation. However, 1336 and 712 RNAs had >1.25-fold significant changes in expression in total and/or polysomal RNA induced by endothelin-1 or insulin, respectively, of which approximately 35% of endothelin-1-responsive and approximately 56% of insulin-responsive transcripts were translationally regulated. Of mRNAs for established proteins recruited to polysomes in response to insulin, 49 were known TOP mRNAs with a further 15 probable/possible TOP mRNAs, but 49 had no identifiable TOP sequences or other consistent features in the 5' untranslated region.
Conclusions: Endothelin-1, rather than insulin, substantially affects global transcript expression to promote cardiomyocyte hypertrophy. Effects on RNA recruitment to polysomes are subtle, with differential effects of endothelin-1 and insulin on specific transcripts. Furthermore, although insulin promotes recruitment of TOP mRNAs to cardiomyocyte polysomes, not all recruited mRNAs are TOP mRNAs.
Figures
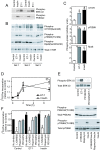
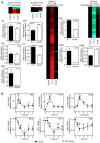
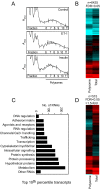
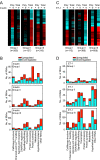
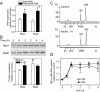
Similar articles
-
Translational activation of 5'-TOP mRNA in pressure overload myocardium.Basic Res Cardiol. 2008 Jan;103(1):41-53. doi: 10.1007/s00395-007-0682-z. Epub 2007 Oct 19. Basic Res Cardiol. 2008. PMID: 17955327
-
Tuberous sclerosis complex proteins 1 and 2 control serum-dependent translation in a TOP-dependent and -independent manner.Mol Cell Biol. 2007 Aug;27(16):5746-64. doi: 10.1128/MCB.02136-06. Epub 2007 Jun 11. Mol Cell Biol. 2007. PMID: 17562867 Free PMC article.
-
Temporal regulation of expression of immediate early and second phase transcripts by endothelin-1 in cardiomyocytes.Genome Biol. 2008;9(2):R32. doi: 10.1186/gb-2008-9-2-r32. Epub 2008 Feb 14. Genome Biol. 2008. PMID: 18275597 Free PMC article.
-
Ribosomal protein S6 kinase from TOP mRNAs to cell size.Prog Mol Biol Transl Sci. 2009;90:109-53. doi: 10.1016/S1877-1173(09)90003-5. Epub 2009 Oct 27. Prog Mol Biol Transl Sci. 2009. PMID: 20374740 Review.
-
Mitogenic and nutritional signals are transduced into translational efficiency of TOP mRNAs.Cold Spring Harb Symp Quant Biol. 2001;66:477-84. doi: 10.1101/sqb.2001.66.477. Cold Spring Harb Symp Quant Biol. 2001. PMID: 12762050 Review. No abstract available.
Cited by
-
Modulation of inflammatory gene expression by the ribotoxin deoxynivalenol involves coordinate regulation of the transcriptome and translatome.Toxicol Sci. 2013 Jan;131(1):153-63. doi: 10.1093/toxsci/kfs266. Epub 2012 Sep 11. Toxicol Sci. 2013. PMID: 22968694 Free PMC article.
-
G Protein-Coupled Receptors As Regulators of Localized Translation: The Forgotten Pathway?Front Endocrinol (Lausanne). 2018 Feb 2;9:17. doi: 10.3389/fendo.2018.00017. eCollection 2018. Front Endocrinol (Lausanne). 2018. PMID: 29456523 Free PMC article. Review.
-
Feedback regulation by Atf3 in the endothelin-1-responsive transcriptome of cardiomyocytes: Egr1 is a principal Atf3 target.Biochem J. 2012 Jun 1;444(2):343-55. doi: 10.1042/BJ20120125. Biochem J. 2012. PMID: 22390138 Free PMC article.
-
The cardiomyocyte "redox rheostat": Redox signalling via the AMPK-mTOR axis and regulation of gene and protein expression balancing survival and death.J Mol Cell Cardiol. 2019 Apr;129:118-129. doi: 10.1016/j.yjmcc.2019.02.006. Epub 2019 Feb 13. J Mol Cell Cardiol. 2019. PMID: 30771309 Free PMC article.
-
Glucose controls co-translation of structurally related mRNAs via the mTOR and eIF2 pathways in human pancreatic beta cells.Front Endocrinol (Lausanne). 2022 Aug 5;13:949097. doi: 10.3389/fendo.2022.949097. eCollection 2022. Front Endocrinol (Lausanne). 2022. PMID: 35992129 Free PMC article.
References
Publication types
MeSH terms
Substances
Grants and funding
LinkOut - more resources
Full Text Sources
Medical