Argonaute quenching and global changes in Dicer homeostasis caused by a pathogen-encoded GW repeat protein
- PMID: 20439431
- PMCID: PMC2861190
- DOI: 10.1101/gad.1908710
Argonaute quenching and global changes in Dicer homeostasis caused by a pathogen-encoded GW repeat protein
Erratum in
-
Corrigendum: Argonaute quenching and global changes in Dicer homeostasis caused by a pathogen-encoded GW repeat protein.Genes Dev. 2018 Apr 1;32(7-8):593-595. doi: 10.1101/gad.314419.118. Genes Dev. 2018. PMID: 29692358 Free PMC article. No abstract available.
Abstract
In plants and invertebrates, viral-derived siRNAs processed by the RNaseIII Dicer guide Argonaute (AGO) proteins as part of antiviral RNA-induced silencing complexes (RISC). As a counterdefense, viruses produce suppressor proteins (VSRs) that inhibit the host silencing machinery, but their mechanisms of action and cellular targets remain largely unknown. Here, we show that the Turnip crinckle virus (TCV) capsid, the P38 protein, acts as a homodimer, or multiples thereof, to mimic host-encoded glycine/tryptophane (GW)-containing proteins normally required for RISC assembly/function in diverse organisms. The P38 GW residues bind directly and specifically to Arabidopsis AGO1, which, in addition to its role in endogenous microRNA-mediated silencing, is identified as a major effector of TCV-derived siRNAs. Point mutations in the P38 GW residues are sufficient to abolish TCV virulence, which is restored in Arabidopsis ago1 hypomorphic mutants, uncovering both physical and genetic interactions between the two proteins. We further show how AGO1 quenching by P38 profoundly impacts the cellular availability of the four Arabidopsis Dicers, uncovering an AGO1-dependent, homeostatic network that functionally connects these factors together. The likely widespread occurrence and expected consequences of GW protein mimicry on host silencing pathways are discussed in the context of innate and adaptive immunity in plants and metazoans.
Figures
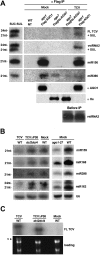
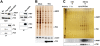
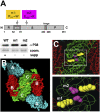
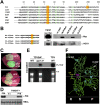
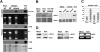
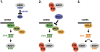
Comment in
-
A viral suppressor protein inhibits host RNA silencing by hooking up with Argonautes.Genes Dev. 2010 May;24(9):853-6. doi: 10.1101/gad.1927310. Genes Dev. 2010. PMID: 20439425 Free PMC article.
Similar articles
-
Requirement for Host RNA-Silencing Components and the Virus-Silencing Suppressor when Second-Site Mutations Compensate for Structural Defects in the 3' Untranslated Region.J Virol. 2015 Nov;89(22):11603-18. doi: 10.1128/JVI.01566-15. Epub 2015 Sep 9. J Virol. 2015. PMID: 26355083 Free PMC article.
-
A viral suppressor protein inhibits host RNA silencing by hooking up with Argonautes.Genes Dev. 2010 May;24(9):853-6. doi: 10.1101/gad.1927310. Genes Dev. 2010. PMID: 20439425 Free PMC article.
-
Molecular modeling and interaction between Arabidopsis sulfite oxidase and the GW motif of Turnip crinkle virus coat protein.Virology. 2020 Dec;551:64-74. doi: 10.1016/j.virol.2020.08.013. Epub 2020 Sep 26. Virology. 2020. PMID: 33038689
-
RNA-based viral immunity initiated by the Dicer family of host immune receptors.Immunol Rev. 2009 Jan;227(1):176-88. doi: 10.1111/j.1600-065X.2008.00722.x. Immunol Rev. 2009. PMID: 19120484 Free PMC article. Review.
-
A structural perspective of the protein-RNA interactions involved in virus-induced RNA silencing and its suppression.Biochim Biophys Acta. 2009 Sep-Oct;1789(9-10):642-52. doi: 10.1016/j.bbagrm.2009.05.006. Epub 2009 Jun 6. Biochim Biophys Acta. 2009. PMID: 19501679 Review.
Cited by
-
Beyond Loading: Functions of Plant ARGONAUTE Proteins.Int J Mol Sci. 2023 Nov 7;24(22):16054. doi: 10.3390/ijms242216054. Int J Mol Sci. 2023. PMID: 38003244 Free PMC article. Review.
-
AGO2: A New Argonaute Compromising Plant Virus Accumulation.Front Plant Sci. 2012 Jan 18;2:112. doi: 10.3389/fpls.2011.00112. eCollection 2011. Front Plant Sci. 2012. PMID: 22639628 Free PMC article.
-
Differential effects of viral silencing suppressors on siRNA and miRNA loading support the existence of two distinct cellular pools of ARGONAUTE1.EMBO J. 2012 May 30;31(11):2553-65. doi: 10.1038/emboj.2012.92. Epub 2012 Apr 24. EMBO J. 2012. PMID: 22531783 Free PMC article.
-
RNA silencing amplification in plants: size matters.Proc Natl Acad Sci U S A. 2010 Aug 24;107(34):14945-6. doi: 10.1073/pnas.1009416107. Epub 2010 Aug 13. Proc Natl Acad Sci U S A. 2010. PMID: 20709960 Free PMC article. No abstract available.
-
Distinct roles of Argonaute in the green alga Chlamydomonas reveal evolutionary conserved mode of miRNA-mediated gene expression.Sci Rep. 2019 Jul 31;9(1):11091. doi: 10.1038/s41598-019-47415-x. Sci Rep. 2019. PMID: 31366981 Free PMC article.
References
-
- Baulcombe D 2004. RNA silencing in plants. Nature 431: 356–363 - PubMed
-
- Bechtold N, Pelletier G 1998. In planta Agrobacterium-mediated transformation of adult Arabidopsis thaliana plants by vacuum infiltration. Methods Mol Biol 82: 259–266 - PubMed
-
- Cabanes D, Dehoux P, Dussurget O, Frangeul L, Cossart P 2002. Surface proteins and the pathogenic potential of Listeria monocytogenes. Trends Microbiol 10: 238–245 - PubMed
Publication types
MeSH terms
Substances
LinkOut - more resources
Full Text Sources
Molecular Biology Databases
Research Materials