Improvement in lipid and protein trafficking in Niemann-Pick C1 cells by correction of a secondary enzyme defect
- PMID: 20412078
- PMCID: PMC3000737
- DOI: 10.1111/j.1600-0854.2010.01046.x
Improvement in lipid and protein trafficking in Niemann-Pick C1 cells by correction of a secondary enzyme defect
Abstract
Different primary lysosomal trafficking defects lead to common alterations in lipid trafficking, suggesting cooperative interactions among lysosomal lipids. However, cellular analysis of the functional consequences of this phenomenon is lacking. As a test case, we studied cells with defective Niemann-Pick C1 (NPC1) protein, a cholesterol trafficking protein whose defect gives rise to lysosomal accumulation of cholesterol and other lipids, leading to NPC disease. NPC1 cells also develop a secondary defect in acid sphingomyelinase (SMase) activity despite a normal acid SMase gene (SMPD1). When acid SMase activity was restored to normal levels in NPC1-deficient CHO cells through SMPD1 transfection, there was a dramatic reduction in lysosomal cholesterol. Two other defects, excess lysosomal bis-(monoacylglycerol) phosphate (BMP) and defective transferrin receptor (TfR) recycling, were also markedly improved. To test its relevance in human cells, the acid SMase activity defect in fibroblasts from NPC1 patients was corrected by SMPD1 transfection or acid SMase enzyme replacement. Both treatments resulted in a dramatic reduction in lysosomal cholesterol. These data show that correcting one aspect of a complex lysosomal lipid storage disease can reduce the cellular consequences even if the primary genetic defect is not corrected.
Figures
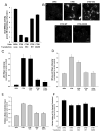
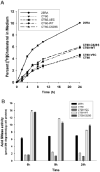
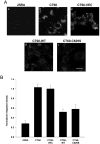
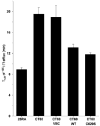
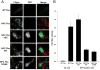
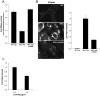
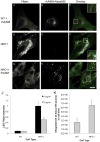
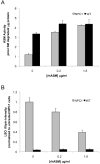
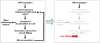
Similar articles
-
Niemann-Pick C1 protein: obligatory roles for N-terminal domains and lysosomal targeting in cholesterol mobilization.Proc Natl Acad Sci U S A. 1999 Feb 2;96(3):805-10. doi: 10.1073/pnas.96.3.805. Proc Natl Acad Sci U S A. 1999. PMID: 9927649 Free PMC article.
-
Cholesterol trapping in Niemann-Pick disease type B fibroblasts can be relieved by expressing the phosphotyrosine binding domain of GULP.J Clin Lipidol. 2013 Mar-Apr;7(2):153-64. doi: 10.1016/j.jacl.2012.02.006. Epub 2012 Feb 22. J Clin Lipidol. 2013. PMID: 23415435
-
The Niemann-Pick C1 protein resides in a vesicular compartment linked to retrograde transport of multiple lysosomal cargo.J Biol Chem. 1999 Apr 2;274(14):9627-35. doi: 10.1074/jbc.274.14.9627. J Biol Chem. 1999. PMID: 10092649
-
Overview of clinical, molecular, and therapeutic features of Niemann-Pick disease (types A, B, and C): Focus on therapeutic approaches.Cell Biochem Funct. 2024 Jun;42(4):e4028. doi: 10.1002/cbf.4028. Cell Biochem Funct. 2024. PMID: 38715125 Review.
-
Niemann-Pick type C mutations cause lipid traffic jam.Traffic. 2000 Mar;1(3):218-25. doi: 10.1034/j.1600-0854.2000.010304.x. Traffic. 2000. PMID: 11208105 Review.
Cited by
-
Abnormal accumulation and recycling of glycoproteins visualized in Niemann-Pick type C cells using the chemical reporter strategy.Proc Natl Acad Sci U S A. 2013 Jun 18;110(25):10207-12. doi: 10.1073/pnas.1221105110. Epub 2013 Jun 3. Proc Natl Acad Sci U S A. 2013. PMID: 23733943 Free PMC article.
-
Identification of Niemann-Pick C1 disease biomarkers through sphingolipid profiling.J Lipid Res. 2013 Oct;54(10):2800-14. doi: 10.1194/jlr.M040618. Epub 2013 Jul 23. J Lipid Res. 2013. PMID: 23881911 Free PMC article.
-
Sensitivity to lysosome-dependent cell death is directly regulated by lysosomal cholesterol content.PLoS One. 2012;7(11):e50262. doi: 10.1371/journal.pone.0050262. Epub 2012 Nov 16. PLoS One. 2012. PMID: 23166840 Free PMC article.
-
Heat shock protein-based therapy as a potential candidate for treating the sphingolipidoses.Sci Transl Med. 2016 Sep 7;8(355):355ra118. doi: 10.1126/scitranslmed.aad9823. Sci Transl Med. 2016. PMID: 27605553 Free PMC article.
-
Anatomically defined neuron-based rescue of neurodegenerative Niemann-Pick type C disorder.J Neurosci. 2011 Mar 23;31(12):4367-78. doi: 10.1523/JNEUROSCI.5981-10.2011. J Neurosci. 2011. PMID: 21430138 Free PMC article.
References
-
- Brady RO. Lysosomal storage diseases. Pharmacol Ther. 1982;19:327–336. - PubMed
-
- Neufeld EF. Lysosomal storage diseases. Annu Rev Biochem. 1991;60:257–280. - PubMed
-
- Puri V, Watanabe R, Dominguez M, Sun X, Wheatley CL, Marks DL, Pagano RE. Cholesterol modulates membrane traffic along the endocytic pathway in sphingolipid-storage diseases. Nat Cell Biol. 1999;1:386–388. - PubMed
-
- Lloyd-Evans E, Morgan AJ, He X, Smith DA, Elliot-Smith E, Sillence DJ, Churchill GC, Schuchman EH, Galione A, Platt FM. Niemann-Pick disease type C1 is a sphingosine storage disease that causes deregulation of lysosomal calcium. Nat Med. 2008;14:1247–1255. - PubMed
-
- Cheruku SR, Xu Z, Dutia R, Lobel P, Storch J. Mechanism of cholesterol transfer from the Niemann-Pick type C2 protein to model membranes supports a role in lysosomal cholesterol transport. J Biol Chem. 2006;281:31594–31604. - PubMed
Publication types
MeSH terms
Substances
Grants and funding
LinkOut - more resources
Full Text Sources
Other Literature Sources
Research Materials
Miscellaneous