Reconstitution of the RIG-I pathway reveals a signaling role of unanchored polyubiquitin chains in innate immunity
- PMID: 20403326
- PMCID: PMC2919214
- DOI: 10.1016/j.cell.2010.03.029
Reconstitution of the RIG-I pathway reveals a signaling role of unanchored polyubiquitin chains in innate immunity
Abstract
RIG-I detects invading viral RNA and activates the transcription factors NF-kappaB and IRF3 through the mitochondrial protein MAVS. Here we show that RNA bearing 5'-triphosphate strongly activates the RIG-I-IRF3 signaling cascade in a reconstituted system composed of RIG-I, mitochondria, and cytosol. Activation of RIG-I requires not only RNA but also polyubiquitin chains linked through lysine 63 (K63) of ubiquitin. RIG-I binds specifically to K63-polyubiquitin chains through its tandem CARD domains in a manner that depends on RNA and ATP. Mutations in the CARD domains that abrogate ubiquitin binding also impair RIG-I activation. Remarkably, unanchored K63-ubiquitin chains, which are not conjugated to any target protein, potently activate RIG-I. These ubiquitin chains function as an endogenous ligand of RIG-I in human cells. Our results delineate the mechanism of RIG-I activation, identify CARD domains as a ubiquitin sensor, and demonstrate that unanchored K63-polyubiquitin chains are signaling molecules in antiviral innate immunity.
Copyright 2010 Elsevier Inc. All rights reserved.
Figures
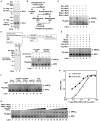
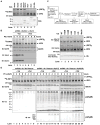
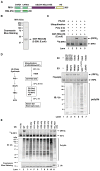
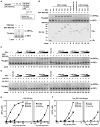
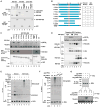
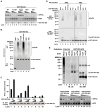
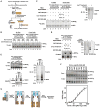
Comment in
-
Ubiquitin gets CARDed.Cell. 2010 Apr 16;141(2):220-2. doi: 10.1016/j.cell.2010.03.047. Cell. 2010. PMID: 20403317
-
Innate immunity: A chain reaction.Nat Rev Immunol. 2010 Jun;10(6):385. doi: 10.1038/nri2783. Nat Rev Immunol. 2010. PMID: 20514677 No abstract available.
Similar articles
-
Activation of duck RIG-I by TRIM25 is independent of anchored ubiquitin.PLoS One. 2014 Jan 23;9(1):e86968. doi: 10.1371/journal.pone.0086968. eCollection 2014. PLoS One. 2014. PMID: 24466302 Free PMC article.
-
MAVS-loaded unanchored Lys63-linked polyubiquitin chains activate the RIG-I-MAVS signaling cascade.Cell Mol Immunol. 2023 Oct;20(10):1186-1202. doi: 10.1038/s41423-023-01065-2. Epub 2023 Aug 15. Cell Mol Immunol. 2023. PMID: 37582970 Free PMC article.
-
Structural and biochemical studies of RIG-I antiviral signaling.Protein Cell. 2013 Feb;4(2):142-54. doi: 10.1007/s13238-012-2088-4. Epub 2012 Dec 20. Protein Cell. 2013. PMID: 23264040 Free PMC article.
-
Ubiquitin-mediated modulation of the cytoplasmic viral RNA sensor RIG-I.J Biochem. 2012 Jan;151(1):5-11. doi: 10.1093/jb/mvr111. Epub 2011 Sep 2. J Biochem. 2012. PMID: 21890623 Review.
-
Recent Advances and Contradictions in the Study of the Individual Roles of Ubiquitin Ligases That Regulate RIG-I-Like Receptor-Mediated Antiviral Innate Immune Responses.Front Immunol. 2020 Jun 24;11:1296. doi: 10.3389/fimmu.2020.01296. eCollection 2020. Front Immunol. 2020. PMID: 32670286 Free PMC article. Review.
Cited by
-
Ubiquitin regulates caspase recruitment domain-mediated signaling by nucleotide-binding oligomerization domain-containing proteins NOD1 and NOD2.J Biol Chem. 2013 Mar 8;288(10):6890-902. doi: 10.1074/jbc.M112.413781. Epub 2013 Jan 8. J Biol Chem. 2013. PMID: 23300079 Free PMC article.
-
RIG-I-like receptor regulation in virus infection and immunity.Curr Opin Virol. 2015 Jun;12:7-14. doi: 10.1016/j.coviro.2015.01.004. Epub 2015 Jan 30. Curr Opin Virol. 2015. PMID: 25644461 Free PMC article. Review.
-
Enterovirus 71 protease 2Apro targets MAVS to inhibit anti-viral type I interferon responses.PLoS Pathog. 2013 Mar;9(3):e1003231. doi: 10.1371/journal.ppat.1003231. Epub 2013 Mar 21. PLoS Pathog. 2013. PMID: 23555247 Free PMC article.
-
Transmissible proteins: expanding the prion heresy.Cell. 2012 May 25;149(5):968-77. doi: 10.1016/j.cell.2012.05.007. Cell. 2012. PMID: 22632966 Free PMC article.
-
Structural insights into the activation of RIG-I, a nanosensor for viral RNAs.EMBO Rep. 2011 Dec 23;13(1):7-8. doi: 10.1038/embor.2011.239. EMBO Rep. 2011. PMID: 22157887 Free PMC article. No abstract available.
References
-
- Akira S, Uematsu S, Takeuchi O. Pathogen recognition and innate immunity. Cell. 2006;124:783–801. - PubMed
-
- Bhoj VG, Chen ZJ. Ubiquitylation in innate and adaptive immunity. Nature. 2009;458:430–437. - PubMed
-
- Cui S, Eisenacher K, Kirchhofer A, Brzozka K, Lammens A, Lammens K, Fujita T, Conzelmann KK, Krug A, Hopfner KP. The C-terminal regulatory domain is the RNA 5′-triphosphate sensor of RIG-I. Mol Cell. 2008;29:169–179. - PubMed
-
- Deng L, Wang C, Spencer E, Yang L, Braun A, You J, Slaughter C, Pickart C, Chen ZJ. Activation of the IkappaB kinase complex by TRAF6 requires a dimeric ubiquitin-conjugating enzyme complex and a unique polyubiquitin chain. Cell. 2000;103:351–361. - PubMed
Publication types
MeSH terms
Substances
Grants and funding
LinkOut - more resources
Full Text Sources
Other Literature Sources
Molecular Biology Databases
Miscellaneous