Transcriptional silencing of {gamma}-globin by BCL11A involves long-range interactions and cooperation with SOX6
- PMID: 20395365
- PMCID: PMC2854393
- DOI: 10.1101/gad.1897310
Transcriptional silencing of {gamma}-globin by BCL11A involves long-range interactions and cooperation with SOX6
Abstract
The developmental switch from human fetal (gamma) to adult (beta) hemoglobin represents a clinically important example of developmental gene regulation. The transcription factor BCL11A is a central mediator of gamma-globin silencing and hemoglobin switching. Here we determine chromatin occupancy of BCL11A at the human beta-globin locus and other genomic regions in vivo by high-resolution chromatin immunoprecipitation (ChIP)-chip analysis. BCL11A binds the upstream locus control region (LCR), epsilon-globin, and the intergenic regions between gamma-globin and delta-globin genes. A chromosome conformation capture (3C) assay shows that BCL11A reconfigures the beta-globin cluster by modulating chromosomal loop formation. We also show that BCL11A and the HMG-box-containing transcription factor SOX6 interact physically and functionally during erythroid maturation. BCL11A and SOX6 co-occupy the human beta-globin cluster along with GATA1, and cooperate in silencing gamma-globin transcription in adult human erythroid progenitors. These findings collectively demonstrate that transcriptional silencing of gamma-globin genes by BCL11A involves long-range interactions and cooperation with SOX6. Our findings provide insight into the mechanism of BCL11A action and new clues for the developmental gene regulatory programs that function at the beta-globin locus.
Figures
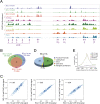
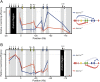
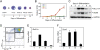
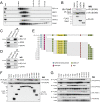
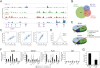
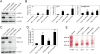
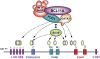
Similar articles
-
Distinct Ldb1/NLI complexes orchestrate γ-globin repression and reactivation through ETO2 in human adult erythroid cells.Blood. 2011 Dec 1;118(23):6200-8. doi: 10.1182/blood-2011-06-363101. Epub 2011 Oct 18. Blood. 2011. PMID: 22010104 Free PMC article.
-
Acyclovir induces fetal hemoglobin via downregulation of γ-globin repressors, BCL11A and SOX6 trans-acting factors.Biochem Pharmacol. 2021 Aug;190:114612. doi: 10.1016/j.bcp.2021.114612. Epub 2021 May 16. Biochem Pharmacol. 2021. PMID: 34010599
-
Binding patterns of BCL11A in the globin and GATA1 loci and characterization of the BCL11A fetal hemoglobin locus.Blood Cells Mol Dis. 2010 Aug 15;45(2):140-6. doi: 10.1016/j.bcmd.2010.05.006. Epub 2010 Jun 12. Blood Cells Mol Dis. 2010. PMID: 20542454
-
Transcriptional Repressor BCL11A in Erythroid Cells.Adv Exp Med Biol. 2024;1459:199-215. doi: 10.1007/978-3-031-62731-6_9. Adv Exp Med Biol. 2024. PMID: 39017845 Review.
-
Epigenetic regulation of fetal globin gene expression in adult erythroid cells.Transl Res. 2015 Jan;165(1):115-25. doi: 10.1016/j.trsl.2014.05.002. Epub 2014 May 11. Transl Res. 2015. PMID: 24880147 Free PMC article. Review.
Cited by
-
NF-Y recruits both transcription activator and repressor to modulate tissue- and developmental stage-specific expression of human γ-globin gene.PLoS One. 2012;7(10):e47175. doi: 10.1371/journal.pone.0047175. Epub 2012 Oct 10. PLoS One. 2012. PMID: 23071749 Free PMC article.
-
Genetics of Sickle Cell-Associated Cardiovascular Disease: An Expert Review with Lessons Learned in Africa.OMICS. 2016 Oct;20(10):581-592. doi: 10.1089/omi.2016.0125. OMICS. 2016. PMID: 27726639 Free PMC article. Review.
-
Featured Article: Modulation of fetal hemoglobin in hereditary persistence of fetal hemoglobin deletion type-2, compared to Sicilian δβ-thalassemia, by BCL11A and SOX6-targeting microRNAs.Exp Biol Med (Maywood). 2017 Feb;242(3):267-274. doi: 10.1177/1535370216668052. Epub 2016 Oct 4. Exp Biol Med (Maywood). 2017. PMID: 27591578 Free PMC article.
-
Combinatorial assembly of developmental stage-specific enhancers controls gene expression programs during human erythropoiesis.Dev Cell. 2012 Oct 16;23(4):796-811. doi: 10.1016/j.devcel.2012.09.003. Epub 2012 Oct 4. Dev Cell. 2012. PMID: 23041383 Free PMC article.
-
Cyclin D3 coordinates the cell cycle during differentiation to regulate erythrocyte size and number.Genes Dev. 2012 Sep 15;26(18):2075-87. doi: 10.1101/gad.197020.112. Epub 2012 Aug 28. Genes Dev. 2012. PMID: 22929040 Free PMC article.
References
-
- Bender MA, Bulger M, Close J, Groudine M 2000. β-Globin gene switching and DNase I sensitivity of the endogenous β-globin locus in mice do not require the locus control region. Mol Cell 5: 387–393 - PubMed
-
- Cantor AB, Orkin SH 2005. Coregulation of GATA factors by the Friend of GATA (FOG) family of multitype zinc finger proteins. Semin Cell Dev Biol 16: 117–128 - PubMed
Publication types
MeSH terms
Substances
Grants and funding
LinkOut - more resources
Full Text Sources
Other Literature Sources