Dissecting the M phase-specific phosphorylation of serine-proline or threonine-proline motifs
- PMID: 20219976
- PMCID: PMC2861607
- DOI: 10.1091/mbc.e09-06-0486
Dissecting the M phase-specific phosphorylation of serine-proline or threonine-proline motifs
Abstract
M phase induction in eukaryotic cell cycles is associated with a burst of protein phosphorylation, primarily at serine or threonine followed by proline (S/TP motif). The mitotic phosphoprotein antibody MPM-2 recognizes a significant subset of mitotically phosphorylated S/TP motifs; however, the required surrounding sequences of and the key kinases that phosphorylate these S/TP motifs remain to be determined. By mapping the mitotic MPM-2 epitopes in Xenopus Cdc25C and characterizing the mitotic MPM-2 epitope kinases in Xenopus oocytes and egg extracts, we have determined that phosphorylation of TP motifs that are surrounded by hydrophobic residues at both -1 and +1 positions plays a dominant role in M phase-associated burst of MPM-2 reactivity. Although mitotic Cdk and MAPK may phosphorylate subsets of these motifs that have a basic residue at the +2 position and a proline residue at the -2 position, respectively, the majority of these motifs that are preferentially phosphorylated in mitosis do not have these features. The M phase-associated burst of MPM-2 reactivity can be induced in Xenopus oocytes and egg extracts in the absence of MAPK or Cdc2 activity. These findings indicate that the M phase-associated burst of MPM-2 reactivity represents a novel type of protein phosphorylation in mitotic regulation.
Figures
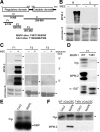
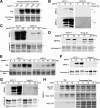
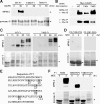
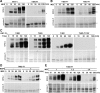
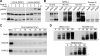
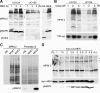
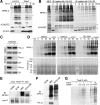
Similar articles
-
MPM-2 epitope sequence is not sufficient for recognition and phosphorylation by ME kinase-H.FEBS Lett. 1997 Aug 25;413(3):417-23. doi: 10.1016/s0014-5793(97)00948-4. FEBS Lett. 1997. PMID: 9303547
-
A phosphatase activity in Xenopus oocyte extracts preferentially dephosphorylates the MPM-2 epitope.FEBS Lett. 1998 Mar 13;424(3):225-33. doi: 10.1016/s0014-5793(98)00158-6. FEBS Lett. 1998. PMID: 9539156
-
Regulation of Cdc25C by ERK-MAP kinases during the G2/M transition.Cell. 2007 Mar 23;128(6):1119-32. doi: 10.1016/j.cell.2006.11.053. Cell. 2007. PMID: 17382881
-
Orchestrating serine/threonine phosphorylation and elucidating downstream effects by short linear motifs.Biochem J. 2022 Jan 14;479(1):1-22. doi: 10.1042/BCJ20200714. Biochem J. 2022. PMID: 34989786 Free PMC article. Review.
-
Pest sequences in nuclear proteins.Int J Biochem. 1993 Apr;25(4):479-82. doi: 10.1016/0020-711x(93)90653-v. Int J Biochem. 1993. PMID: 8096823 Review.
Cited by
-
Determinants for activation of the atypical AGC kinase Greatwall during M phase entry.Mol Cell Biol. 2012 Apr;32(8):1337-53. doi: 10.1128/MCB.06525-11. Epub 2012 Feb 21. Mol Cell Biol. 2012. PMID: 22354989 Free PMC article.
-
Revisiting the multisite phosphorylation that produces the M-phase supershift of key mitotic regulators.Mol Biol Cell. 2022 Oct 1;33(12):ar115. doi: 10.1091/mbc.E22-04-0118. Epub 2022 Aug 17. Mol Biol Cell. 2022. PMID: 35976701 Free PMC article.
-
Phosphoproteomic Profiling Identifies Aberrant Activation of Integrin Signaling in Aggressive Non-Type Bladder Carcinoma.J Clin Med. 2019 May 17;8(5):703. doi: 10.3390/jcm8050703. J Clin Med. 2019. PMID: 31108958 Free PMC article.
-
Phosphorylation-Dependent Activation of the ESCRT Function of ALIX in Cytokinetic Abscission and Retroviral Budding.Dev Cell. 2016 Feb 8;36(3):331-43. doi: 10.1016/j.devcel.2016.01.001. Dev Cell. 2016. PMID: 26859355 Free PMC article.
-
Bioinformatics Analysis of Global Proteomic and Phosphoproteomic Data Sets Revealed Activation of NEK2 and AURKA in Cancers.Biomolecules. 2020 Feb 4;10(2):237. doi: 10.3390/biom10020237. Biomolecules. 2020. PMID: 32033228 Free PMC article.
References
-
- Alvarez E., Northwood I. C., Gonzalez F. A., Latour D. A., Seth A., Abate C., Curran T., Davis R. J. Pro-Leu-Ser/Thr-Pro is a consensus primary sequence for substrate protein phosphorylation. Characterization of the phosphorylation of c-myc and c-jun proteins by an epidermal growth factor receptor threonine 669 protein kinase. J. Biol. Chem. 1991;266:15277–15285. - PubMed
-
- Brittle A. L., Ohkura H. Centrosome maturation: Aurora lights the way to the poles. Curr. Biol. 2005;15:R880–R882. - PubMed
-
- Campbell L. E., Proud C. G. Differing substrate specificities of members of the DYRK family of arginine-directed protein kinases. FEBS Lett. 2002;510:31–36. - PubMed
-
- Cano E., Mahadevan L. C. Parallel signal processing among mammalian MAPKs. Trends Biochem. Sci. 1995;20:117–122. - PubMed
-
- Che S., El-Hodiri H. M., Wu C. F., Nelman-Gonzalez M., Weil M. M., Etkin L. D., Clark R. B., Kuang J. Identification and cloning of xp95, a putative signal transduction protein in Xenopus oocytes. J. Biol. Chem. 1999;274:5522–5531. - PubMed
Publication types
MeSH terms
Substances
Grants and funding
LinkOut - more resources
Full Text Sources
Research Materials
Miscellaneous