Mitotic homologous recombination maintains genomic stability and suppresses tumorigenesis
- PMID: 20177395
- PMCID: PMC3261768
- DOI: 10.1038/nrm2851
Mitotic homologous recombination maintains genomic stability and suppresses tumorigenesis
Abstract
Mitotic homologous recombination promotes genome stability through the precise repair of DNA double-strand breaks and other lesions that are encountered during normal cellular metabolism and from exogenous insults. As a result, homologous recombination repair is essential during proliferative stages in development and during somatic cell renewal in adults to protect against cell death and mutagenic outcomes from DNA damage. Mutations in mammalian genes encoding homologous recombination proteins, including BRCA1, BRCA2 and PALB2, are associated with developmental abnormalities and tumorigenesis. Recent advances have provided a clearer understanding of the connections between these proteins and of the key steps of homologous recombination and DNA strand exchange.
Conflict of interest statement
The authors declare no competing financial interests.
Figures
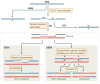
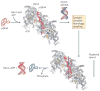
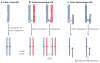
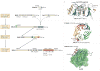
Similar articles
-
Drosophila brca2 is required for mitotic and meiotic DNA repair and efficient activation of the meiotic recombination checkpoint.PLoS Genet. 2008 Feb;4(2):e31. doi: 10.1371/journal.pgen.0040031. PLoS Genet. 2008. PMID: 18266476 Free PMC article.
-
Double-strand break repair and homologous recombination in Schizosaccharomyces pombe.Yeast. 2006 Oct 15;23(13):963-76. doi: 10.1002/yea.1414. Yeast. 2006. PMID: 17072889 Review.
-
BRIT1/MCPH1 is essential for mitotic and meiotic recombination DNA repair and maintaining genomic stability in mice.PLoS Genet. 2010 Jan 22;6(1):e1000826. doi: 10.1371/journal.pgen.1000826. PLoS Genet. 2010. PMID: 20107607 Free PMC article.
-
Error-Prone Repair of DNA Double-Strand Breaks.J Cell Physiol. 2016 Jan;231(1):15-24. doi: 10.1002/jcp.25053. J Cell Physiol. 2016. PMID: 26033759 Free PMC article. Review.
-
Bacterial DNA repair genes and their eukaryotic homologues: 5. The role of recombination in DNA repair and genome stability.Acta Biochim Pol. 2007;54(3):483-94. Epub 2007 Sep 23. Acta Biochim Pol. 2007. PMID: 17893749 Review.
Cited by
-
A marker-free genetic manipulation method for Glaesserella parasuis strains developed by alternately culturing transformants at 37°C and 30°C.BMC Biotechnol. 2024 Sep 3;24(1):60. doi: 10.1186/s12896-024-00887-w. BMC Biotechnol. 2024. PMID: 39227838 Free PMC article.
-
Functional Validation of Rare Human Genetic Variants Involved in Homologous Recombination Using Saccharomyces cerevisiae.PLoS One. 2015 May 4;10(5):e0124152. doi: 10.1371/journal.pone.0124152. eCollection 2015. PLoS One. 2015. PMID: 25938495 Free PMC article.
-
DNA Polymerase θ: A Unique Multifunctional End-Joining Machine.Genes (Basel). 2016 Sep 21;7(9):67. doi: 10.3390/genes7090067. Genes (Basel). 2016. PMID: 27657134 Free PMC article. Review.
-
Single strand DNA binding proteins 1 and 2 protect newly replicated telomeres.Cell Res. 2013 May;23(5):705-19. doi: 10.1038/cr.2013.31. Epub 2013 Mar 5. Cell Res. 2013. PMID: 23459151 Free PMC article.
-
BRCA1 and TP53 codeficiency causes a PARP inhibitor-sensitive erythroproliferative neoplasm.JCI Insight. 2022 Dec 22;7(24):e158257. doi: 10.1172/jci.insight.158257. JCI Insight. 2022. PMID: 36346676 Free PMC article.
References
-
- Hoeijmakers JH. DNA damage, aging, and cancer. N Engl J Med. 2009;361:1475–1485. - PubMed
-
- Negrini S, Gorgoulis VG, Halazonetis TD. Genomic instability — an evolving hallmark of cancer. Nature Rev Mol Cell Biol. 2010;11:220–228. - PubMed
-
- Aquilera A, Rothstein R. Molecular Genetics of Recombination. Springer; Berlin Germany: 2007.
-
- Haber JE. Partners and pathways repairing a double-strand break. Trends Genet. 2000;16:259–264. - PubMed
Publication types
MeSH terms
Grants and funding
LinkOut - more resources
Full Text Sources
Other Literature Sources
Research Materials
Miscellaneous