DHP-derivative and low oxygen tension effectively induces human adipose stromal cell reprogramming
- PMID: 20161735
- PMCID: PMC2817727
- DOI: 10.1371/journal.pone.0009026
DHP-derivative and low oxygen tension effectively induces human adipose stromal cell reprogramming
Abstract
Background and methods: In this study, we utilized a combination of low oxygen tension and a novel anti-oxidant, 4-(3,4-dihydroxy-phenyl)-derivative (DHP-d) to directly induce adipose tissue stromal cells (ATSC) to de-differentiate into more primitive stem cells. De-differentiated ATSCs was overexpress stemness genes, Rex-1, Oct-4, Sox-2, and Nanog. Additionally, demethylation of the regulatory regions of Rex-1, stemnesses, and HIF1alpha and scavenging of reactive oxygen species were finally resulted in an improved stem cell behavior of de-differentiate ATSC (de-ATSC). Proliferation activity of ATSCs after dedifferentiation was induced by REX1, Oct4, and JAK/STAT3 directly or indirectly. De-ATSCs showed increased migration activity that mediated by P38/JUNK and ERK phosphorylation. Moreover, regenerative efficacy of de-ATSC engrafted spinal cord-injured rats and chemical-induced diabetes animals were significantly restored their functions.
Conclusions/significance: Our stem cell remodeling system may provide a good model which would provide insight into the molecular mechanisms underlying ATSC proliferation and transdifferentiation. Also, these multipotent stem cells can be harvested may provide us with a valuable reservoir of primitive and autologous stem cells for use in a broad spectrum of regenerative cell-based disease therapy.
Conflict of interest statement
Figures
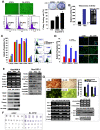
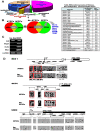
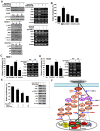
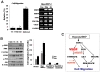
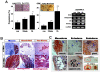
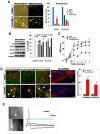
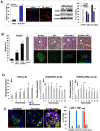
Similar articles
-
IFATS collection: Selenium induces improvement of stem cell behaviors in human adipose-tissue stromal cells via SAPK/JNK and stemness acting signals.Stem Cells. 2008 Oct;26(10):2724-34. doi: 10.1634/stemcells.2008-0184. Epub 2008 Jun 26. Stem Cells. 2008. Retraction in: Stem Cells. 2013 Dec;31(12):2848. doi: 10.1002/stem.1591. PMID: 18583539 Retracted.
-
Regulation of adipose tissue stromal cells behaviors by endogenic Oct4 expression control.PLoS One. 2009 Sep 24;4(9):e7166. doi: 10.1371/journal.pone.0007166. PLoS One. 2009. PMID: 19777066 Free PMC article.
-
Cytoplasmic extracts from adipose tissue stromal cells alleviates secondary damage by modulating apoptosis and promotes functional recovery following spinal cord injury.Brain Pathol. 2007 Jul;17(3):263-75. doi: 10.1111/j.1750-3639.2007.00070.x. Epub 2007 Apr 23. Brain Pathol. 2007. PMID: 17465991 Free PMC article.
-
Adipose tissue-derived stromal cells as a novel option for regenerative cell therapy.J Atheroscler Thromb. 2006 Apr;13(2):77-81. doi: 10.5551/jat.13.77. J Atheroscler Thromb. 2006. PMID: 16733294 Review.
-
Bone marrow stromal cells for spinal cord repair: a challenge for contemporary neurobiology.Histol Histopathol. 2009 Jan;24(1):107-16. doi: 10.14670/HH-24.107. Histol Histopathol. 2009. PMID: 19012250 Review.
Cited by
-
Generation of reactive oxygen species in adipose-derived stem cells: friend or foe?Expert Opin Ther Targets. 2011 Nov;15(11):1297-306. doi: 10.1517/14728222.2011.628315. Epub 2011 Oct 10. Expert Opin Ther Targets. 2011. PMID: 21981031 Free PMC article. Review.
-
Hypoxia Suppresses Spontaneous Mineralization and Osteogenic Differentiation of Mesenchymal Stem Cells via IGFBP3 Up-Regulation.Int J Mol Sci. 2016 Aug 24;17(9):1389. doi: 10.3390/ijms17091389. Int J Mol Sci. 2016. PMID: 27563882 Free PMC article.
-
Adipogenesis of adipose-derived stem cells may be regulated via the cytoskeleton at physiological oxygen levels in vitro.Stem Cell Res Ther. 2013 Jul 9;4(4):79. doi: 10.1186/scrt230. Stem Cell Res Ther. 2013. PMID: 23838354 Free PMC article.
-
An Overview of the Mechanism behind Excessive Volume of Pericardial Fat in Heart Failure.J Obes Metab Syndr. 2023 Dec 30;32(4):322-329. doi: 10.7570/jomes23042. Epub 2023 Dec 1. J Obes Metab Syndr. 2023. PMID: 38036419 Free PMC article. Review.
-
Primary involvement of NADPH oxidase 4 in hypoxia-induced generation of reactive oxygen species in adipose-derived stem cells.Stem Cells Dev. 2012 Aug 10;21(12):2212-21. doi: 10.1089/scd.2011.0561. Epub 2012 Feb 3. Stem Cells Dev. 2012. PMID: 22181007 Free PMC article.
References
-
- Goodell MA. Stem-cell “plasticity”: befuddled by the muddle. Curr opin hematol. 2003;10:208–13. - PubMed
-
- Wagers AJ, Weissman IL. Plasticity of adult stem cells. Cell. 2004;116:639–48. - PubMed
-
- Grafi G. How cells dedifferentiate: a lesson from plants. Dev Biol. 2004;268:1–6. - PubMed
-
- Takebe I, Labib G, Melchers G. Regeneration of whole plants from isolated mesophyll protoplasts of tobacco. Naturwissenschaften. 1971;58:318–320.
-
- Valente O, Tao W, Verbelen JP. Auxins and cytokinins control DNA endoreduplication and deduplication in single cells of tobacco. Plant Sci. 1998;134:207–215.
Publication types
MeSH terms
Substances
LinkOut - more resources
Full Text Sources
Research Materials
Miscellaneous