Ultrahigh-throughput screening in drop-based microfluidics for directed evolution
- PMID: 20142500
- PMCID: PMC2840095
- DOI: 10.1073/pnas.0910781107
Ultrahigh-throughput screening in drop-based microfluidics for directed evolution
Erratum in
- Proc Natl Acad Sci U S A. 2010 Apr 6;107(14):6550
Abstract
The explosive growth in our knowledge of genomes, proteomes, and metabolomes is driving ever-increasing fundamental understanding of the biochemistry of life, enabling qualitatively new studies of complex biological systems and their evolution. This knowledge also drives modern biotechnologies, such as molecular engineering and synthetic biology, which have enormous potential to address urgent problems, including developing potent new drugs and providing environmentally friendly energy. Many of these studies, however, are ultimately limited by their need for even-higher-throughput measurements of biochemical reactions. We present a general ultrahigh-throughput screening platform using drop-based microfluidics that overcomes these limitations and revolutionizes both the scale and speed of screening. We use aqueous drops dispersed in oil as picoliter-volume reaction vessels and screen them at rates of thousands per second. To demonstrate its power, we apply the system to directed evolution, identifying new mutants of the enzyme horseradish peroxidase exhibiting catalytic rates more than 10 times faster than their parent, which is already a very efficient enzyme. We exploit the ultrahigh throughput to use an initial purifying selection that removes inactive mutants; we identify approximately 100 variants comparable in activity to the parent from an initial population of approximately 10(7). After a second generation of mutagenesis and high-stringency screening, we identify several significantly improved mutants, some approaching diffusion-limited efficiency. In total, we screen approximately 10(8) individual enzyme reactions in only 10 h, using < 150 microL of total reagent volume; compared to state-of-the-art robotic screening systems, we perform the entire assay with a 1,000-fold increase in speed and a 1-million-fold reduction in cost.
Conflict of interest statement
Patent applications that include some of the ideas described in this manuscript have been filed by Harvard University, the Medical Research Council, UK, and the University of Strasbourg. Should these institutions receive revenues as a result of licensing these patents, the authors are entitled to receive payments through the respective Inventor’s Rewards Schemes. J.J.A. is founder of Fluid Discovery, which uses ultrahigh-throughput screening methods derived from ideas described herein. A.D.G. and D.A.W. are also founders of Raindance Technologies, which has licensed some of these patent applications.
Figures
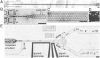
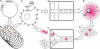
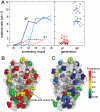
Similar articles
-
Controlled Continuous Evolution of Enzymatic Activity Screened at Ultrahigh Throughput Using Drop-Based Microfluidics.Angew Chem Int Ed Engl. 2023 Jun 12;62(24):e202303112. doi: 10.1002/anie.202303112. Epub 2023 May 3. Angew Chem Int Ed Engl. 2023. PMID: 37019845
-
A completely in vitro ultrahigh-throughput droplet-based microfluidic screening system for protein engineering and directed evolution.Lab Chip. 2012 Mar 7;12(5):882-91. doi: 10.1039/c2lc21035e. Epub 2012 Jan 26. Lab Chip. 2012. PMID: 22277990
-
Efficient molecular evolution to generate enantioselective enzymes using a dual-channel microfluidic droplet screening platform.Nat Commun. 2018 Mar 12;9(1):1030. doi: 10.1038/s41467-018-03492-6. Nat Commun. 2018. PMID: 29531246 Free PMC article.
-
Droplet Microfluidics and Directed Evolution of Enzymes: An Intertwined Journey.Angew Chem Int Ed Engl. 2021 Nov 8;60(46):24368-24387. doi: 10.1002/anie.202016154. Epub 2021 Jul 16. Angew Chem Int Ed Engl. 2021. PMID: 33539653 Free PMC article. Review.
-
Directed Evolution in Drops: Molecular Aspects and Applications.ACS Synth Biol. 2021 Nov 19;10(11):2772-2783. doi: 10.1021/acssynbio.1c00313. Epub 2021 Oct 22. ACS Synth Biol. 2021. PMID: 34677942 Free PMC article. Review.
Cited by
-
Targeted Single-Cell RNA and DNA Sequencing With Fluorescence-Activated Droplet Merger.Anal Chem. 2020 Nov 3;92(21):14616-14623. doi: 10.1021/acs.analchem.0c03059. Epub 2020 Oct 13. Anal Chem. 2020. PMID: 33049138 Free PMC article.
-
High-throughput screening of filamentous fungi using nanoliter-range droplet-based microfluidics.Sci Rep. 2016 Jun 7;6:27223. doi: 10.1038/srep27223. Sci Rep. 2016. PMID: 27270141 Free PMC article.
-
Computational Design of the β-Sheet Surface of a Red Fluorescent Protein Allows Control of Protein Oligomerization.PLoS One. 2015 Jun 15;10(6):e0130582. doi: 10.1371/journal.pone.0130582. eCollection 2015. PLoS One. 2015. PMID: 26075618 Free PMC article.
-
Tailored Fluorosurfactants through Controlled/Living Radical Polymerization for Highly Stable Microfluidic Droplet Generation.Angew Chem Int Ed Engl. 2024 Jan 15;63(3):e202315552. doi: 10.1002/anie.202315552. Epub 2023 Dec 12. Angew Chem Int Ed Engl. 2024. PMID: 38038248 Free PMC article.
-
Label-free isolation and deposition of single bacterial cells from heterogeneous samples for clonal culturing.Sci Rep. 2016 Sep 6;6:32837. doi: 10.1038/srep32837. Sci Rep. 2016. PMID: 27596612 Free PMC article.
References
-
- Joyce AR, Palsson BO. The model organism as a system: Integrating ‘omics’ data sets. Nat Rev Mol Cell Biol. 2006;7:198–210. - PubMed
-
- Carter PJ. Potent antibody therapeutics by design. Nat Rev Immunol. 2006;6:343–357. - PubMed
-
- Bershtein S, Tawfik DS. Advances in laboratory evolution of enzymes. Curr Opin Chem Biol. 2008;12:151–158. - PubMed
-
- Keasling JD. Synthetic biology for synthetic chemistry. ACS Chem Biol. 2008;3:64–76. - PubMed
-
- Peisajovich SG, Tawfik DS. Protein engineers turned evolutionists. Nat Methods. 2007;4:991–994. - PubMed
Publication types
MeSH terms
Substances
LinkOut - more resources
Full Text Sources
Other Literature Sources