Basic principles and concepts underlying recent advances in magnetic resonance imaging of the developing brain
- PMID: 20109968
- PMCID: PMC2887750
- DOI: 10.1053/j.semperi.2009.10.001
Basic principles and concepts underlying recent advances in magnetic resonance imaging of the developing brain
Abstract
Over the last decade, magnetic resonance (MR) imaging has become an essential tool in the evaluation of both in vivo human brain development and perinatal brain injury. Recent technology including MR-compatible neonatal incubators, neonatal head coils, advanced MR pulse sequences, and 3-T field strength magnets allow high-quality MR imaging studies to be performed on sick neonates. This article will review basic principles and concepts underlying recent advances in MR spectroscopy, diffusion, perfusion, and volumetric MR imaging. These techniques provide quantitative assessment and novel insight of both brain development and brain injury in the immature brain. Knowledge of normal developmental changes in quantitative MR values is also essential to interpret pathologic cases.
(c) 2010 Elsevier Inc. All rights reserved.
Figures
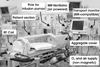
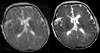
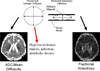
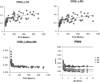
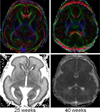
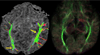
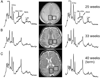
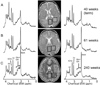
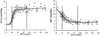
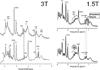
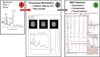
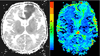
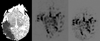
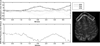
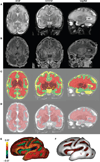
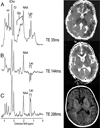
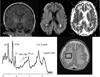
Similar articles
-
Advances in magnetic resonance neuroimaging techniques in the evaluation of neonatal encephalopathy.Top Magn Reson Imaging. 2007 Feb;18(1):3-29. doi: 10.1097/RMR.0b013e318093e6c7. Top Magn Reson Imaging. 2007. PMID: 17607141 Review.
-
MRI evaluation and safety in the developing brain.Semin Perinatol. 2015 Mar;39(2):73-104. doi: 10.1053/j.semperi.2015.01.002. Epub 2015 Mar 3. Semin Perinatol. 2015. PMID: 25743582 Free PMC article. Review.
-
Advanced MR techniques in the term-born neonate with perinatal brain injury.Semin Fetal Neonatal Med. 2005 Oct;10(5):445-60. doi: 10.1016/j.siny.2005.05.005. Semin Fetal Neonatal Med. 2005. PMID: 15979420 Review.
-
Understanding brain injury and neurodevelopmental disabilities in the preterm infant: the evolving role of advanced magnetic resonance imaging.Semin Perinatol. 2010 Feb;34(1):57-66. doi: 10.1053/j.semperi.2009.10.006. Semin Perinatol. 2010. PMID: 20109973 Free PMC article. Review.
-
MR imaging of the neonatal brain.Neuroimaging Clin N Am. 2006 Feb;16(1):117-35, viii-ix. doi: 10.1016/j.nic.2005.10.003. Neuroimaging Clin N Am. 2006. PMID: 16543088 Review.
Cited by
-
The pattern of brain metabolism in chronic steno-occlusive cerebral artery disease.Ann Transl Med. 2022 Sep;10(18):1003. doi: 10.21037/atm-22-3993. Ann Transl Med. 2022. PMID: 36267716 Free PMC article.
-
Developmental synergy between thalamic structure and interhemispheric connectivity in the visual system of preterm infants.Neuroimage Clin. 2015 Jun 4;8:462-72. doi: 10.1016/j.nicl.2015.05.014. eCollection 2015. Neuroimage Clin. 2015. PMID: 26106571 Free PMC article.
-
Anterior cingulate and frontal lobe white matter spectroscopy in early childhood of former very LBW premature infants.Pediatr Res. 2011 Mar;69(3):224-9. doi: 10.1203/PDR.0b013e3182091d52. Pediatr Res. 2011. PMID: 21135758 Free PMC article.
-
Diffusion Tensor Imaging of White Matter in Children Born from Preeclamptic Gestations.AJNR Am J Neuroradiol. 2017 Apr;38(4):801-806. doi: 10.3174/ajnr.A5064. Epub 2017 Jan 26. AJNR Am J Neuroradiol. 2017. PMID: 28126749 Free PMC article.
-
Altered brain metabolism contributes to executive function deficits in school-aged children born very preterm.Pediatr Res. 2020 Nov;88(5):739-748. doi: 10.1038/s41390-020-1024-1. Epub 2020 Jun 26. Pediatr Res. 2020. PMID: 32590836 Free PMC article.
References
-
- Ferriero DM. Neonatal brain injury. N Engl J Med. 2004;351:1985–1995. - PubMed
-
- Bluml S, Friedlich P, Erberich S, et al. MR imaging of newborns by using an MR-compatible incubator with integrated radiofrequency coils: initial experience. Radiology. 2004;231:594–601. - PubMed
-
- Dumoulin CL, Rohling KW, Piel JE, et al. Magnetic resonance imaging compatible neonate incubator. Concepts Magn Reson. 2002;15:117–128.
-
- Mathur AM, Neil JL, McKinstry RC, et al. Transport, monitoring, and successful MR imaging in unsedated neonates. Pediatr Rad. 2008;38:260–264. - PubMed
-
- Forbes KPN, Pipe JG, Bird CR, et al. PROPELLER MRI: clinical testing of a novel technique for quantification and compensation of head motion. J Magn Reson Imaging. 2001;14:215–222. - PubMed
Publication types
MeSH terms
Grants and funding
LinkOut - more resources
Full Text Sources
Medical