Retrieval of the vacuolar H-ATPase from phagosomes revealed by live cell imaging
- PMID: 20052281
- PMCID: PMC2796722
- DOI: 10.1371/journal.pone.0008585
Retrieval of the vacuolar H-ATPase from phagosomes revealed by live cell imaging
Abstract
Background: The vacuolar H+-ATPase, or V-ATPase, is a highly-conserved multi-subunit enzyme that transports protons across membranes at the expense of ATP. The resulting proton gradient serves many essential functions, among them energizing transport of small molecules such as neurotransmitters, and acidifying organelles such as endosomes. The enzyme is not present in the plasma membrane from which a phagosome is formed, but is rapidly delivered by fusion with endosomes that already bear the V-ATPase in their membranes. Similarly, the enzyme is thought to be retrieved from phagosome membranes prior to exocytosis of indigestible material, although that process has not been directly visualized.
Methodology: To monitor trafficking of the V-ATPase in the phagocytic pathway of Dictyostelium discoideum, we fed the cells yeast, large particles that maintain their shape during trafficking. To track pH changes, we conjugated the yeast with fluorescein isothiocyanate. Cells were labeled with VatM-GFP, a fluorescently-tagged transmembrane subunit of the V-ATPase, in parallel with stage-specific endosomal markers or in combination with mRFP-tagged cytoskeletal proteins.
Principal findings: We find that the V-ATPase is commonly retrieved from the phagosome membrane by vesiculation shortly before exocytosis. However, if the cells are kept in confined spaces, a bulky phagosome may be exocytosed prematurely. In this event, a large V-ATPase-rich vacuole coated with actin typically separates from the acidic phagosome shortly before exocytosis. This vacuole is propelled by an actin tail and soon acquires the properties of an early endosome, revealing an unexpected mechanism for rapid recycling of the V-ATPase. Any V-ATPase that reaches the plasma membrane is also promptly retrieved.
Conclusions/significance: Thus, live cell microscopy has revealed both a usual route and alternative means of recycling the V-ATPase in the endocytic pathway.
Conflict of interest statement
Figures
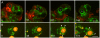
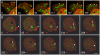
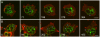
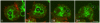
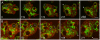
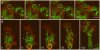
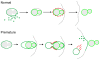
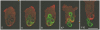
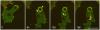
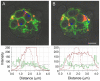
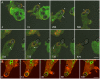
Similar articles
-
Dynamics of the vacuolar H(+)-ATPase in the contractile vacuole complex and the endosomal pathway of Dictyostelium cells.J Cell Sci. 2002 Jul 15;115(Pt 14):2893-905. doi: 10.1242/jcs.115.14.2893. J Cell Sci. 2002. PMID: 12082150
-
Heterogeneous modes of uptake for latex beads revealed through live cell imaging of phagocytes expressing a probe for phosphatidylinositol-(3,4,5)-trisphosphate and phosphatidylinositol-(3,4)-bisphosphate.Cell Motil Cytoskeleton. 2008 Sep;65(9):721-33. doi: 10.1002/cm.20293. Cell Motil Cytoskeleton. 2008. PMID: 18561186
-
Direct recruitment of H+-ATPase from lysosomes for phagosomal acidification.J Cell Sci. 2009 Jul 15;122(Pt 14):2504-13. doi: 10.1242/jcs.050443. Epub 2009 Jun 23. J Cell Sci. 2009. PMID: 19549681
-
Vacuolar and plasma membrane proton-adenosinetriphosphatases.Physiol Rev. 1999 Apr;79(2):361-85. doi: 10.1152/physrev.1999.79.2.361. Physiol Rev. 1999. PMID: 10221984 Review.
-
Vacuolar ATPase in phago(lyso)some biology.Int J Med Microbiol. 2018 Jan;308(1):58-67. doi: 10.1016/j.ijmm.2017.08.007. Epub 2017 Sep 1. Int J Med Microbiol. 2018. PMID: 28867521 Review.
Cited by
-
Dissection of Francisella-Host Cell Interactions in Dictyostelium discoideum.Appl Environ Microbiol. 2015 Dec 28;82(5):1586-1598. doi: 10.1128/AEM.02950-15. Appl Environ Microbiol. 2015. PMID: 26712555 Free PMC article.
-
Legionella pneumophila infection is enhanced in a RacH-null mutant of Dictyostelium.Commun Integr Biol. 2011 Mar;4(2):194-7. doi: 10.4161/cib.4.2.14381. Commun Integr Biol. 2011. PMID: 21655438 Free PMC article.
-
Serine/Threonine Protein Phosphatase PstP of Mycobacterium tuberculosis Is Necessary for Accurate Cell Division and Survival of Pathogen.J Biol Chem. 2016 Nov 11;291(46):24215-24230. doi: 10.1074/jbc.M116.754531. Epub 2016 Oct 7. J Biol Chem. 2016. PMID: 27758870 Free PMC article.
-
Nramp1 and NrampB Contribute to Resistance against Francisella in Dictyostelium.Front Cell Infect Microbiol. 2017 Jun 21;7:282. doi: 10.3389/fcimb.2017.00282. eCollection 2017. Front Cell Infect Microbiol. 2017. PMID: 28680861 Free PMC article.
-
A functional connection of Dictyostelium paracaspase with the contractile vacuole and a possible partner of the vacuolar proton ATPase.J Biosci. 2013 Sep;38(3):509-21. doi: 10.1007/s12038-013-9338-3. J Biosci. 2013. PMID: 23938384
References
-
- Breton S, Brown D. New insights into the regulation of V-ATPase-dependent proton secretion. Am J Physiol Renal Physiol. 2007;292:F1–10. - PubMed
-
- Saroussi S, Nelson N. Vacuolar H(+)-ATPase-an enzyme for all seasons. Pflugers Arch. 2009;457:581–587. - PubMed
-
- Nolta KV, Rodriguez-Paris JM, Steck TL. Analysis of successive endocytic compartments isolated from Dictyostelium discoideum by magnetic fractionation. Biochim Biophys Acta. 1994;1224:237–246. - PubMed
Publication types
MeSH terms
Substances
LinkOut - more resources
Full Text Sources
Molecular Biology Databases